Unconventional Superconductivity Robust Against Impurities in a Newly Discovered Kagome Lattice Superconductor
PI of Joint-use project: K. Hashimoto
Host lab: Uwatoko Group
Host lab: Uwatoko Group
One of the strategies in the search for quantum phenomena such as non-trivial magnetism and superconductivity is to focus on the symmetry of crystal structures of materials. The kagome lattice consisting of corner-sharing triangles and hexagonal holes (Fig. 1(a)) has attracted much attention because materials with kagome lattice structures exhibit various exotic properties, such as quantum spin liquids, Dirac/Weyl physics, and unconventional superconductivity, driven by its geometry. In this context, the discovery of superconductivity in AV3Sb5 (A = K, Rb, Cs) with a perfect kagome lattice in 2020 has received significant attention [1]. In these materials, charge density wave (CDW) orders with a so-called (inverse) star-of-David pattern of electronic hopping have been observed above the superconducting transition temperature (Fig. 1(b)) [2]. Very interestingly, it has been reported that these CDW states break time-reversal and rotational symmetries. In addition, high-pressure studies have revealed that the CDW phase is suppressed by applying pressure, accompanied by the emergence of a superconducting dome, indicating the close relationship between the CDW and superconductivity [3,4]. Therefore, one of the most crucial unresolved issues is identifying the symmetry of the superconductivity that develops inside the CDW phase. However, the mechanism of superconductivity in AV3Sb5 and its relationship with the CDW state have remained elusive [2].
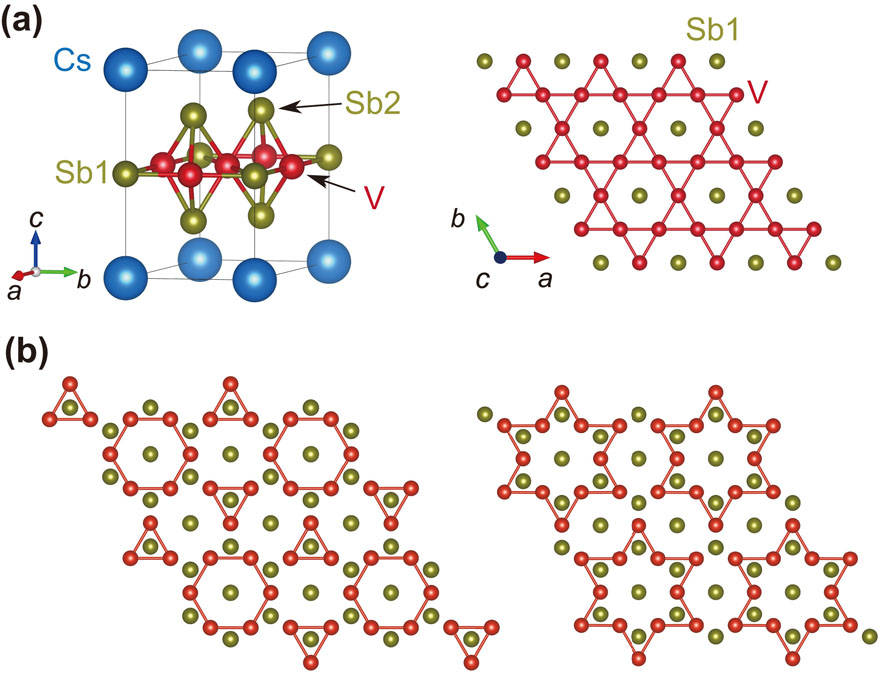
Fig. 1. (a) Crystal structure of CsV3Sb5. V-Sb1 plane viewed from the c-axis direction (right). Whereas the V atoms form a two-dimensional kagome network, the Sb1 atoms are located at the hexagonal centers.(b) CDW pattern in CsV3Sb5. V and Sb1 atoms form the so-called star of David (right) and inverse star of David (left) patterns. These patterns alternate in the interplane direction.
Here, we focus on the effect of impurities on the superconducting state to elucidate the mechanism of superconductivity in AV3Sb5. Based on the Bardeen-Cooper-Schrieffer (BCS) theory, which is the standard theory of superconductivity, the conventional superconducting state arises from the pairing of two electrons induced by electron-phonon interactions. In this case, the introduction of nonmagnetic impurities generally has little effect on the superconducting transition temperature (Tc) and the superconducting gap structure [5]. However, it is well known that unconventional superconducting states, as observed in high-Tc cuprates and heavy-fermion superconductors, are significantly suppressed by nonmagnetic impurities.
In this study, we systematically investigated the impurity effects of CsV3Sb5 on the superconducting transition temperature and the superconducting gap structure [6]. The introduction of non-magnetic impurities into crystals was achieved by using electron irradiation, enabling precise control of the impurity concentration in the CsV3Sb5 single-crystal samples. We examined the change in the superconducting transition temperature by measuring the resistivity of the impurity-controlled samples at ambient and high pressure. We also performed magnetic penetration depth measurements, which reflect the superconducting gap structure. As a result, we found that the superconducting transition temperature of CsV3Sb5 is rapidly suppressed at a low impurity concentration but starts to saturate at moderate impurity concentrations (Fig. 2(a)). This behavior differs from those of both conventional BCS superconductors and unconventional superconductors such as high-Tc cuprates. In addition, our magnetic penetration depth measurements reveal that with increasing impurities, an anisotropic fully-gapped state changes to an isotropic full-gap state without showing impurity-induced Andreev bound states, which excludes any of sign-changing symmetries (Fig. 2(b)). Furthermore, transport measurements under pressure show that the double superconducting dome in the pressure-temperature phase diagram survives against sufficient impurities (Fig. 3). These results support that CsV3Sb5 is a non-chiral, anisotropic s-wave superconductor with no sign change both at ambient and under pressure. The present findings support a new type of unconventional superconductivity originated from bond-order fluctuations in the CDW state of CsV3Sb5, where the gap function is non-sign-changing s-wave [7].
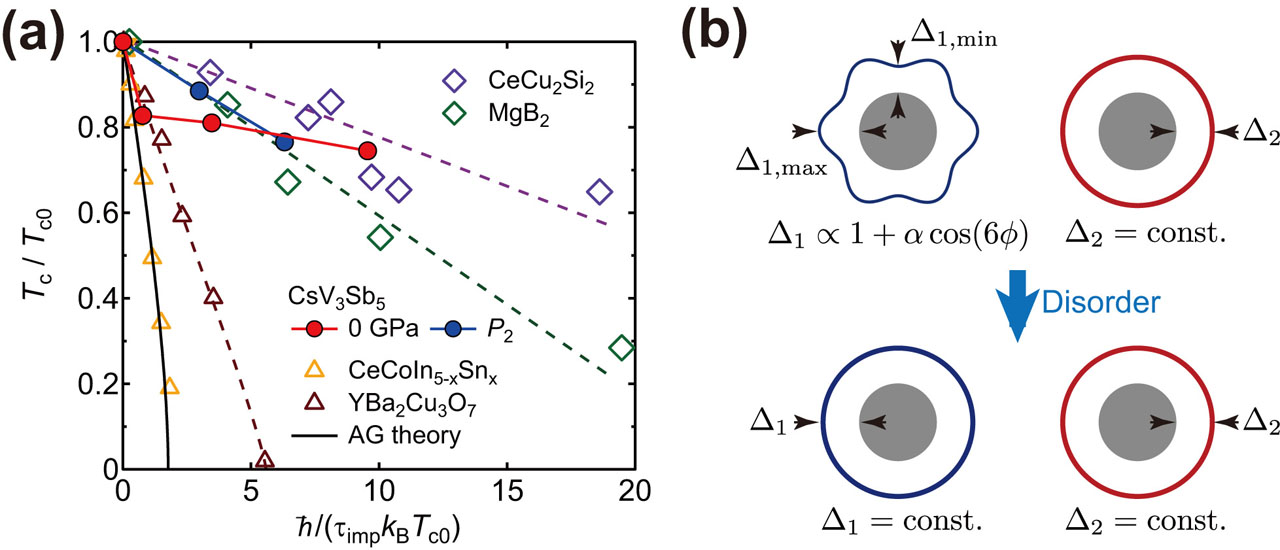
Fig. 2. (a) Suppression of the superconducting transition temperature Tc in CsV3Sb5 as a function of pair breaking parameter (corresponding to the amount of impurities). Red circles represent the transition temperature under ambient pressure (0 GPa), and blue circles represent the transition temperature at P2. (b) Changes in the superconducting gap structure in CsV3Sb5 by impurities. The gap structure is anisotropic before the introduction of impurities, but as the impurity concentration is increased, the gap size averages out and eventually the two gaps become isotropic and almost the same size. This behavior is consistent with the theoretical expectation in the bond-order fluctuation superconducting mechanism [6].
Our present study will pave the way to understanding the unique electronic and superconducting states in kagome lattice materials.
References
- [1] B. R. Ortiz et al., Phys. Rev. Lett. 125, 247002 (2020).
- [2] T. Neupert et al., Nat. Phys. 18, 137 (2022).
- [3] K. Y. Chen et al., Phys. Rev. Lett. 126, 247001 (2021).
- [4] R. Gupta et al., Commun. Phys. 5, 232 (2022).
- [5] P. W. Anderson, J. Phys. Chem. Solids 11, 26 (1959).
- [6] M. Roppongi et al., Nature Communications 14, 667 (2023).
- [7] R. Tazai et al., Sci. Adv. 8, eabl4108 (2022).