Rise of Nonlinear Soft X-Ray Spectroscopy
I. Matsuda Group
X-ray spectroscopy has been a powerful technique to probe electronic states in materials. The light-matter interaction is based on a linear-response but it becomes non-linear when the intensity of incident light is significantly high. Recently, developments of X-ray free electron laser (XFEL) have led to our observation of the second harmonic generation, one of the well known non-linear optical phenomena, with soft X-ray (SX-SHG)[1]. A SX-SHG event occurs only when the inversion symmetry of the targeted system is broken and the signal is element-specific. These unique characters have pushed to apply SX-SHG for spectroscopy of materials science. However, it is often difficult to acquire a pure SX-SHG beam from a sample when the intensity is as small as second-harmonic radiation, generated on off-axis at the undulators. Such signal contamination is harmful for discussing genuine properties of SHG from any target sample. To overcome the situation, we developed and installed a new ellipsometry method for soft X-ray SHG experiment, as shown in Fig.1, to suppress the contribution of second-harmonic light from the XFEL beamline [2]. By the collaboration with RIKEN, we have also developed an experimental chamber of SX-SHG and opened for the SACLA users. We have successfully detected the broken inversion symmetry in the polar metal phase of LiOsO3 with an enhanced feature above the Li K-edge that reflects the degree of Li atom displacement [3]. In a noncentrosymmetric crystal of LiNbO3, elemental contributions by lithium and niobium were apparently distinguished by energy dependent SX-SHG measurements [4]. We have also probed the lithium mobility at a solid electrolyte surface to develop the Li-ion battery [5].
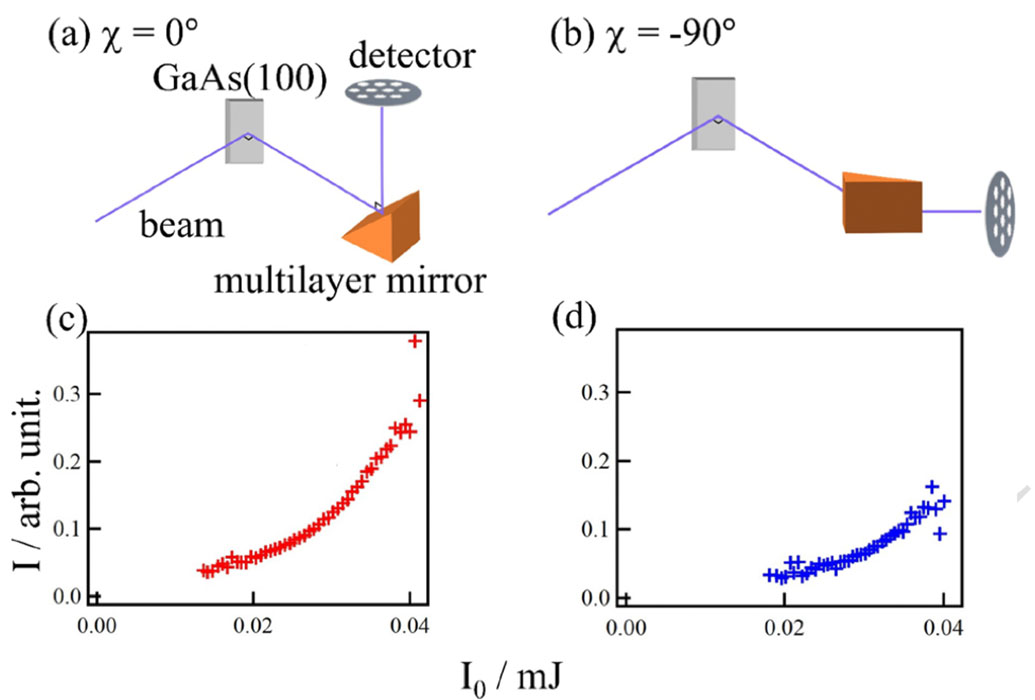
Fig. 1. Soft X-ray SHG data of GaAs(100) taken at the As M absorption edge, recorded with the ellipsometry method. The incident photon (I0) beam was set at hν = 75 eV and the SHG signal (I) was detected at 2hν = 150 eV. The measurement geometries for the ellipsometry rotation angles at (a) χ = 0° and (b) −90°. Intensity curves of the SHG signals are shown in (c) and (d) that were taken by (a) and (b), respectively.
These research have applied the novel measurement method for investigation of crystallographic or electronic properties. The sensitivity to magnetism has remained elusive. Though a magnetic moment as an axial vector does not break the bulk inversion symmetry, it lowers the symmetry at interfaces, and thus should make additional contributions to SHG. While X-ray linear spectroscopies such as X-ray magnetic circular dichroism are useful to element-selectively study the magnetic properties of a material, it averages inevitably information over a certain depth along the incident light. To meet the demand of frontier technology, such as spintronics, that utilizes magnetic materials with complicated multilayered structure, it is, thus, useful to develop an element-selective method that can exclusively probe interface magnetism. We developed a magnetization induced SHG for soft X-ray (SX-MSHG) measurement system and made a demonstration for an Fe-based multilayer samples with broken inversion symmetry [6]. At the Fe M-edge resonance condition, we observed, as shown in Fig. 2, a clear dependence of SX-MSHG on the direction of applied magnetic field, even though the field was along the hard magnetization axis and thus the magnetization was only a small fraction of the saturation value. The results indicate high sensitivity of the measurement likely due to inner-shell resonance. By further deepening the understanding of its unique characteristics, SX-MSHG could be devel ped into a solid technique to element-selectively probe interface magnetism.
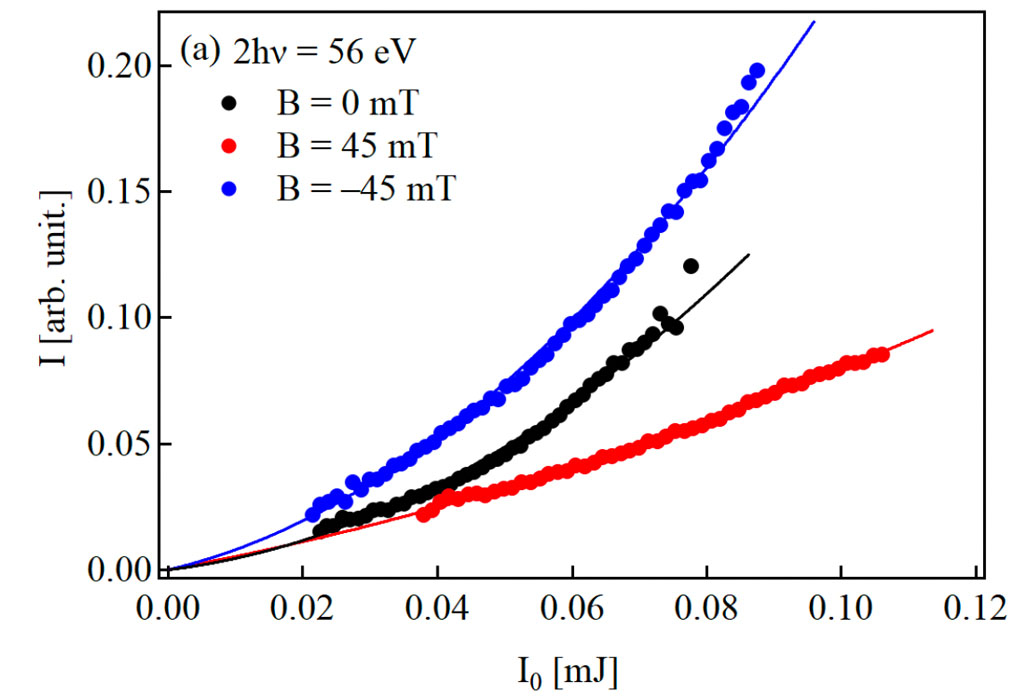
Fig. 2. SX- magnetization induced SHG (MSHG) from the [Au/Fe/MgO]4 sample. 2hν intensity plot with respect to the incident beam intensity I0 at 2hν = 56 eV under magnetic field as indicated. Photon energy of 56 eV corresponds to the Fe M-edge resonance. Solid curves represent the results of fitting with nonlinear curves.
Through the technical efforts, research of the nonlinear optical effect in the soft X-ray region has evolved into a method of nonlinear soft X-ray spectroscopy. The unique characters of both the interface- and element-selectivity are useful for unveiling electronic and magnetic properties of the complicated compounds that provide various functionalities. Nonlinear X-ray spectroscopy is expected to become one of the standard analysis methods for materials science.
References
- [1] Sh. Yamamoto, T. Omi, H. Akai, Y. Kubota, Y. Takahashi, Y. Suzuki, Y. Hirata, K. Yamamoto, R. Yukawa, K. Horiba, H. Yumoto, T. Koyama, H. Ohashi, S. Owada, K. Tono, M. Yabashi, E. Shigemasa, S. Yamamoto, M. Kotsugi, H. Wadati, H. Kumigashira, T. Arima, S. Shin, and I. Matsuda, Phys. Rev. Lett. 120, 223902 (2018).
- [2] T. Sumi, M. Horio, T. Senoo, T. Wada, Y. Tsujikawa, X. Zhang, P. Manset, M. Araki, Y. Hirata, W. S. Drisdell, J. W. Freeland, A. Amado, M. Zuerch, Y. Kubota, S. Owada, K. Tono, M. Yabashi, C. P. Schwartz, and I. Matsuda, e-J. Surf. Sci. Nanotechnol. 20, 25 (2022).
- [3] E. Berger, S. Jamnuch, C. B. Uzundal, C. Woodahl, H. Padmanabhan, A. Amado, P. Manset, Y. Hirata, Y. Kubota, S. Owada, K. Tono, M. Yabashi, C. Wang, Y. Shi, V. Gopalan, C. P. Schwartz, W. S. Drisdell, I. Matsuda, J. W. Freeland, T. A. Pascal, and M. Zuerch, Nano Letters 21, 6095 (2021).
- [4] C. B. Uzundal, S. Jamnuch, E. Berger, C. Woodahl, P. Manset, Y. Hirata, T. Sumi, A. Amado, H. Akai, Y. Kubota, S. Owada, K. Tono, M. Yabashi, J. W. Freeland, C. P. Schwartz, W. S. Drisdell, I. Matsuda, T. A. Pascal, A. Zong, and M. Zuerch, Phys. Rev. Lett. 127, 237402 (2021).
- [5] C. Woodahl, S. Jamnuch, A. Amado, C. B. Uzundal, E. Berger, P. Manset, Y. Zhu, Y. Li, D. D. Fong, J. G. Connell, Y. Hirata, Y. Kubota, S. Owada, K. Tono, M. Yabashi, S. Tepavcevic, I. Matsuda, W. S. Drisdell, C. P. Schwartz, J. W. Freeland, T. A. Pascal, A. Zong, and M. Zuerch, Nat. Mater. (2023). https://doi.org/10.1038/s41563-023-01535-y
- [6] T. Sumi, M. Horio, T. Senoo, Y. Kubota, G. Yamaguchi, T. Wada, M. Miyamoto, K. Yamaguchi, Y. Tsujikawa, Y. Sato, M. Niibe, Y. Hirata, Y. Miyauchi, D. Oshima, T. Kato, S. Owada, K. Tono, M. Yabashi, and I. Matsuda, Appl. Phys. Lett. 122, 171601 (2023).