High Field Quantum Phase in the Chromium Spinel Oxide HgCr2O4
PI of Joint-use project: S. KimuraHost lab: Kindo and Kohama Groups
The spin nematic state, which has long been discussed for magnets with frustrated interactions, is a spin analog of the nematic state in a liquid crystal. In this unconventional magnetic state, the fluctuations of spins, behaving like rod- or disk-shaped molecules, spontaneously select a preferred direction without conventional magnetic order. In this study, we have confirmed a possible spin nematic order in the chromium spinel oxide HgCr2O4 This compound is known as a highly frustrated pylochlore antiferromagnet with significant spin-lattice coupling. In fact, the wide half-magnetization plateau, which was stabilized by the lattice distortion, was reported in HgCr2O4 [1]. The magnetization process in the chromium spinel oxides has been accounted for the classical model with the bilinear and biquadratic interactions, derived by integrating out the lattice degrees of freedom [2]. However, the result of previous high field magnetization measurements indicated the existence of a phase, which cannot be explained by the classical model, just below the saturation field Hc4 [3]. From the subsequent theory, this unknown phase was suggested to be the spin nematic phase, which is stabilized by the interplay of the spin-lattice coupling, geometrical spin frustration and quantum fluctuation [4]. To gain thermodynamic evidence for the existence of the magnetic ordering in the phase just below Hc4, we have performed the specific heat and the magnetocaloric effect measurements under pulsed high magnetic fields up to 42 T [5]. Figure 1 shows the results of the magnetocaloric effect measurements. The sharp dips at Hc3 ~ 37 T and Hc4 ~ 40 T in the magnetocaloric effect under the quasi-isothermal condition unambiguously exhibit the magnetic phase for Hc3 < H < Hc4, whereas the fact that only a small kink is observed at Hc3 under the quasiadiabatic condition indicates that the phase between Hc3 and Hc4 is gapless. From a peak of the specific heat at 38.5 K, we have also confirmed the ordering for Hc3 < H < Hc4. From these thermodynamic measurements, we have obtained the magnetic field and temperature phase diagram, shown in Fig. 2. In the Heisenberg-type pyrochlore lattice magnet, the lowest-energy magnon states in a fully spin-polarized region above the saturation field are localized with flat dispersions owing to the geometrical configuration of the lattice. Therefore, the hopping of the single lowest-energy magnon is prohibited, thereby preventing kinetic energy gain. Meanwhile the spin-lattice coupling in HgCr2O4 brings the effective biquadratic interaction in Eq. (1). This biquadratic interaction contains a term (Si−)2(Sj+)2, which induces hopping of a two-spin flipped state on a Cr3+ site to the nearest neighbor sites. Because of this hopping term, the two-magnon bound state becomes stable and has a lower energy than two independent localized magnons in the fully polarized spin state. Decreasing the magnetic field in the fully polarized spin state causes softening of the two-magnon bound state. Then, the bound state touches to the ground state at the saturation field, resulting in its condensation, which leads to the spin nematic state. According to the calculation, the spin-component distribution, of which the projection to the plane perpendicular to the magnetic field has an ellipsoid shape, is aligned owing to the spin nematic order, thereby breaking the rotational symmetry around field. From our experimental results that suggest the existence of the gapless magnetic ordered phase between Hc3 and Hc4, we consider that such a spin nematic order most probably appears in HgCr2O4. The existence of an unknown magnetic phase just below the saturation was also reported for other chromium spinel oxides, CdCr2O4 and ZnCr2O4 from high-field experiments It is possible that the appearance of the spin nematic phase is of universal nature in chromium spinel oxides.
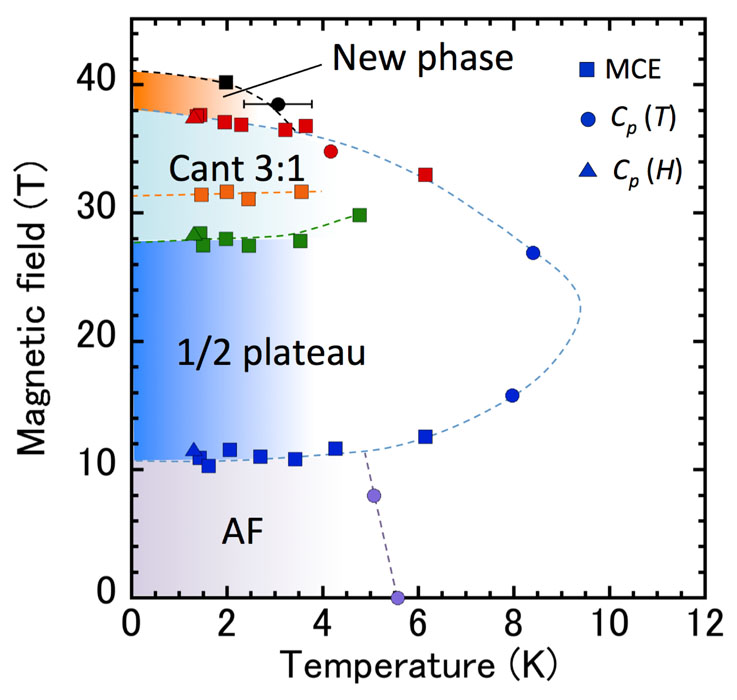
Fig. 2. Magnetic field and temperature phase diagram of HgCr2O4, obtained from thermodynamic experiments. Squares, circles, and triangles are the transition points obtained from the magnetocaloric effect, temperature, and field dependencies of the specific heat, respectively. Dashed curves are drawn as guides for the eyes.
References
- [1] H. Ueda, H. Mitamura, T. Goto, and Y. Ueda, Phys. Rev. B 73, 094415 (2006).
- [2] K. Penc, N. Shannon, and H. Shiba, Phys. Rev. Lett. 93, 197203 (2004).
- [3] S. Kimura, M. Hagiwara, T. Takeuchi, H. Yamaguchi, H. Ueda, Y. Ueda, and K. Kindo, Phys. Rev. B 83, 214401 (2011).
- [4] E. Takata, T. Momoi, and M. Oshikawa, arXiv:1510.02373.
- [5] S. Kimura, S. Imajo, M. Gen, T. Momoi, M. Hagiwara, H. Ueda, and Y. Kohama, Phys. Rev. B 105, L180405 (2022).