Detecting Electron-Phonon Coupling During Photoinduced Phase Transition
Okazaki and Itatani Groups
Photoinduced phase transitions have been intensively studied owing to their potential capabilities to control a material of interest in an ultrafast manner, which can induce exotic phases unable to be attained at equilibrium. As materials of interest, strongly correlated electron systems provide very fruitful playgrounds because they exhibit very rich phases owing to intertwined couplings between multiple degrees of freedom including charge, orbital, spin, and lattice, where photoexcitation is a very promising way to control their physical properties. Recently, we revealed the photoinduced insulator-to-metal transitions (IMTs) in Ta2NiSe5 by using time- and angle-resolved photoemission spectroscopy (TARPES) as schematically shown in Fig. 1(a). Figures 1(b)-1(e) are TARPES spectra of Ta2NiSe5 in each delay time, and Figs. 1(f)-1(h) show differential images of TARPES, where red and blue regions correspond to increasing and decreasing intensity, respectively. One can notice that the photo-induced semimetallic bands shown as red regions in Figs. 1(f)-1(h) cross the Fermi level (EF). However, the key mechanisms are still under debate, and it is currently a central issue as to how the couplings between the electron, lattice, and spin degrees of freedom are evolving during the photoinduced phase transition.
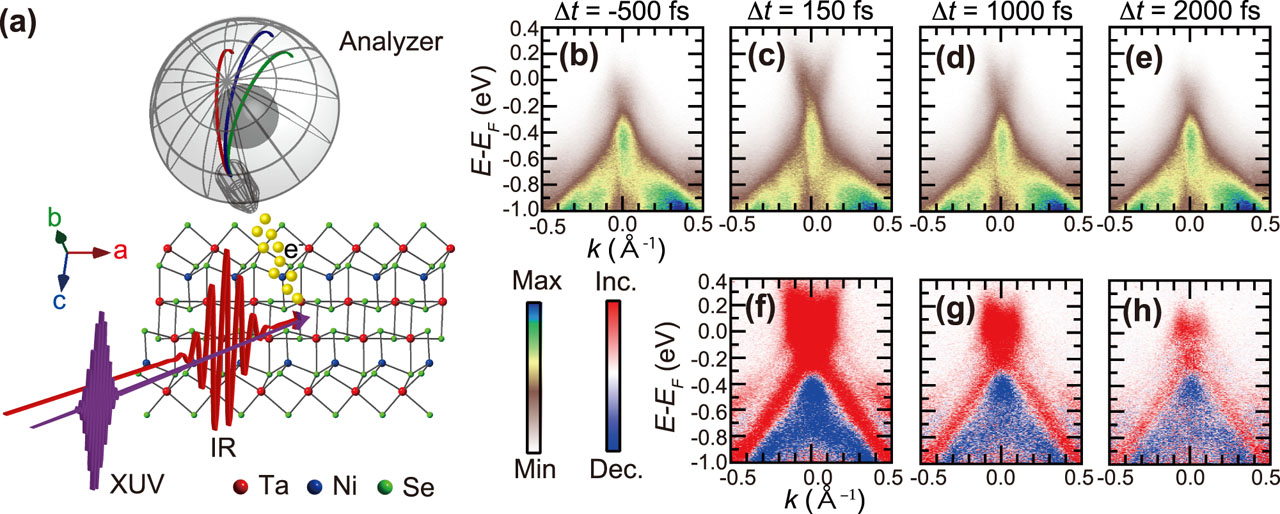
Fig. 1. (a) Schematic illustration of time- and angle-resolved photoemission spectroscopy (TARPES), as applied to Ta2NiSe5. The pump pulse is infrared light, whereas the probe pulse is extreme ultraviolet light produced by high harmonic generation. Photoelectrons are detected by a hemispherical electron analyzer. (b)–(e) TARPES spectra of Ta2NiSe5. The delay time between the pump and probe is indicated in each panel. (f)–(h) Differential images of TARPES. Red and blue points represent increasing and decreasing photoemission intensity, respectively.
In order to reveal the underlying mechanism in terms of the electron-phonon couplings, we mapped out the frequency-dependent intensity of the Fourier components in the energy and momentum space, which we call frequency-domain angle-resolved photoemission spectroscopy (FDARPES). Figures 2(a)–2(e) show the FDARPES spectra corresponding to the frequencies of 1, 2, 3, 3.75, and 4 THz, respectively. To see each phonon mode associated with the FDARPES spectra, we performed the ab initio calculations. First, we calculated the band structure of Ta2NiSe5 based on the density functional theory within a generalized gradient approximation (GGA), and confirmed that the results can overall reproduce the photoemission results as shown in Fig. 2(f). Then, we employed the calculations to obtain the phonon modes based on the density functional perturbation theory. The calculated phonon modes corresponding to the 2- and 3-THz modes are shown in Figs. 2(g) and 2(h). Noticeably, the FDARPES spectra exhibit distinctively different behaviors depending on the frequency, which demonstrates that each phonon mode is selectively coupled to the specific electronic bands. Particularly, the 2-THz phonon mode has the strongest signal around EF, where it consists of a mixture of Ta 5d and Se 4p orbitals, and this signature is responsible for the collapse of the excitonic insulating phase. In other words, the 2-THz phonon mode is revealed to be the most strongly coupled to the emergent photoinduced electronic bands crossing EF. Furthermore, we proceeded to compare each FDARPES spectra with the band dispersions before and after photoexcitation. We found that the FDARPES spectrum at 1 THz matches the band dispersions after photoexcitation better than that before photoexcitation as shown in Fig. 2(a), while the FDARPES spectrum at 3 THz is closer to the band dispersions before photoexcitation as shown in Fig. 2(c). This means that both the semimetallic and semiconducting bands are coexistent in the transient state, and suggests the strong electron-phonon couplings for 1-THz and 3-THz phonon modes are associated with the semimetallic and semiconducting bands, respectively. Moreover, this finding demonstrates that, whereas the semimetallic and semiconducting states coexist after photoexciation, the FDARPES method can selectively reveal the coupling of each phonon mode to semimetallic and semiconducting states by the frequency-domain analysis.
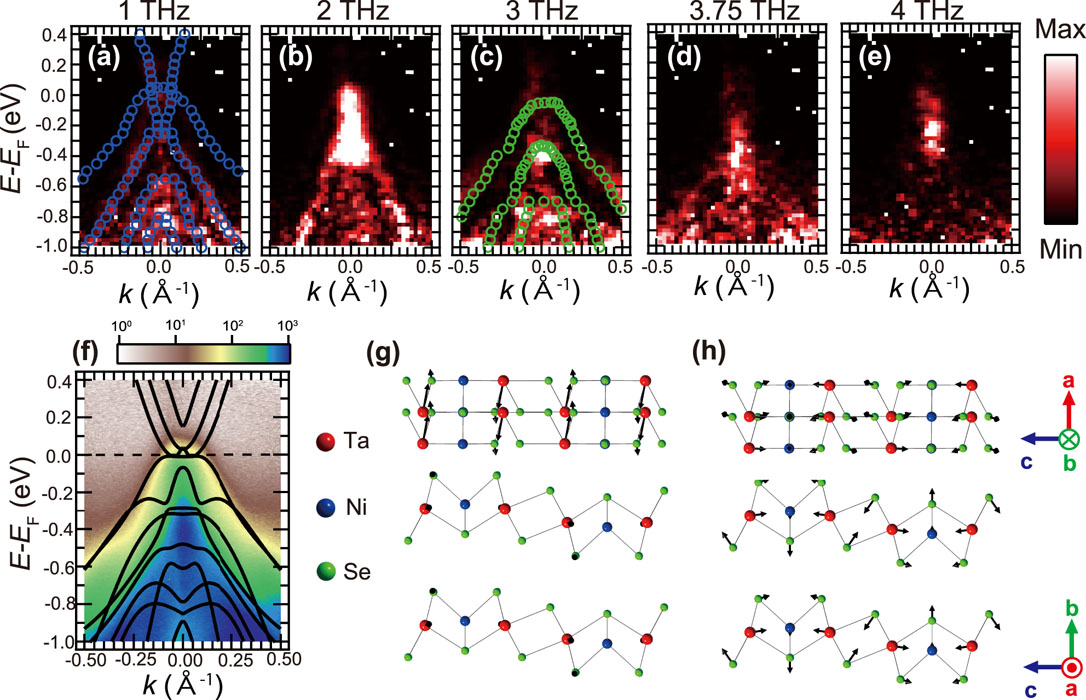
Fig. 2. (a)–(e) Frequency-domain angle-resolved photoemission spectroscopy (FDARPES) spectra shown as frequency-dependent intensities of the oscillation components as a function of energy and momentum. The peak positions in the TARPES spectra after and before photoexcitation are plotted as blue and green circles in (a) and (c), respectively. (f) Calculated band structure of Ta2NiSe5 based on the density functional theory within a generalized gradient approximation (GGA) superimposed onto the photoemission spectrum before pump excitation. (g), (h) Calculated phonon modes corresponding to (b) and (c), respectively.
References
- [1] T. Suzuki, Y. Shinohara, Y. Lu, M. Watanabe, J. Xu, K. L. Ishikawa, H. Takagi, M. Nohara, N. Katayama, H. Sawa, M. Fujisawa, T. Kanai, J. Itatani, T. Mizokawa, S. Shin, and K. Okazaki, Phys. Rev. B 103, L121105 (2021).