Magnetic Field-Induced Phenomena in the Unconventional Superconductor UTe2
Tokunaga Group
Unconventional superconductivity in UTe2 is one of the hot topics in strongly correlated electron systems. The spin-triplet superconductivity is very likely because of its extremely high superconducting (SC) critical fields. Since its discovery three years ago, many experimental and theoretical works with intense competition revealed novel phenomena realized in UTe2 [1]. External parameters, such as pressure and magnetic field (H), strongly affect superconductivity and magnetism in UTe2. One of the intriguing phenomena induced by applied H is the reinforcement and reemergence of SC phases, which are sensitive to the H directions [2]. When the H applied along a hard magnetization b axis, the H reinforces the SC transition above 15 T. With increasing H, the superconducting transition temperature Tsc increases and is suppressed above μ0Hm ~ 35 T, where a first-order metamagnetic transition takes place (see Fig. 1(b)) [3]. More surprisingly, the H along near the [011] direction induces another SC phase above Hm [2]. Thus, the metamagnetic transition strongly affects the SC transition. However, their relationship and the origin of metamagnetism leave many mysteries. Using state-of-the-art pulsed-high-field experiments developed in the International MegaGauss Science Laboratory at the ISSP, we have studied H-induced phenomena in UTe2.
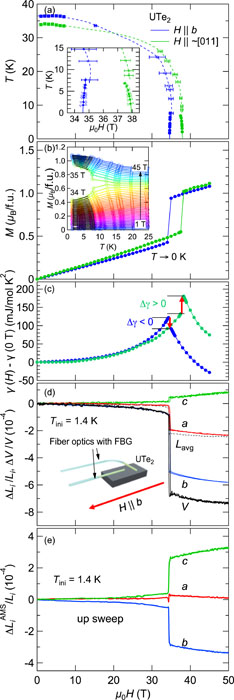
Fig. 1. (a)Phase diagrams of UTe2 for H || b and ~[011]. The inset zooms near Hm. The filled (open) circles and squares indicate Hm (crossover) and Tχmax, respectively. Magnetic field dependences of (b)magnetization extrapolated to 0 K, (c)electronic specific heat coefficient derived by thermodynamic relations, (d)linear and volume magnetostriction at Tini = 1.4 K and (e)anisotropic magnetostriction at Tini = 1.4 K.
In order to address the relationship between metamagnetic and SC transition, we revealed the mass enhancement as a function of the magnetic field and its direction [4]. In general, the larger the density of state, the higher Tsc is. Field dependence of the electronic specific heat coefficient, γ (H), is derived thermodynamically by analyzing the T dependence of magnetization (M) at constant fields and phase diagram. Since the magnetocaloric effect (MCE) is significant [5], the sample temperature varies during the H sweep. To account for the MCE, we measure the sample T and M simultaneously using field-insensitive capacitance thermometers developed by Miyake et al. [6]. This method is also potent in determining the magnetic phase diagram more precisely.
The inset of Fig. 1 (b) shows the M (T) curves at constant H. On approaching Hm, T decreases significantly. This M (T, H) evaluation is the first attempt and a considerable breakthrough in the pulsed-field experiments. The sign change in dM/dT across Hm indicates the existence of a peak of γ (H) at Hm from Maxwell’s relation. The γ (H) should change discontinuously across Hm because of the first-order transition. We solved this issue by employing Clausius-Clapeyron’s equation. As shown in Fig. 1(a), dHm/dT changes in its sign from positive for H || b to negative for H || ~[011]. This change means that the negative Δγ (H) crossing HHm for H || b changes to positive for H || ~[011] [see Fig. 1(c)]. The negative (positive) Δγ (H) for H || b (~[011]) is consistent with the suppression (reemergence) of SC at Hm. These findings hint at understanding the origin of the SC phases varying with the field directions.
Since the metamagnetic transition is of the first order, the volume at least should change across Hm. Thus, we tried to reveal volume/structural change, namely lattice instability, at Hm through the magnetostriction [7]. We performed magnetostriction measurements using the optical fiber with fiber-Bragg-grating and the optical filter method developed in Ikeda et al. [8]. The inset of Fig. 1 (d) is a schematic drawing of the setup for the magnetostriction measurements. The bare fiber optics were glued directly on the sample parallel and perpendicular to the field direction along the b axis, as in Ref. [9]. This method enables us to obtain the longitudinal and transverse magnetostriction simultaneously, the first example in pulsed fields.
ΔLi/Li’s (i = a, b, c) show anisotropic H dependencies and change discontinuously at Hm, as expected for the first-order transition (Fig. 1 (d)). The volume magnetostriction ΔV/V, i.e., a summation of ΔLi/Li, shows a discontinuous change of –5.9 × 10-4 at Hm. Knowing ΔV and ΔM at Hm, we can estimate pressure (p) dependence of Hm from Clausius-Clapeyron’s equation. The Hm(p) obtained by the thermodynamic quantities are in good agreement with the Hm(p) determined experimentally, confirming the reliability of our experiments. An important finding here is the anisotropic magnetostriction (AMS) defined as ΔLiAMS/Li = ΔLi/Li –ΔLavg/Lavg (the averaged linear magnetostrcition ΔLavg/Lavg= ΔV/3V). For the isotropic case, the AMS is small. Interestingly, the strong anisotropy appears in the bc plane, while ΔLaAMS/La is very small. Thus, the metamagnetic transition in UTe2 involves the lattice instability, which plays a crucial role in the SC phases near Hm. We also suggest the possible valence instability in terms of the itinerant-localized duality picture of the uranium magnetism [7].
Without experimental developments, the fascinated H-induced phenomena in UTe2 are not entirely solved. In other words, UTe2 is our good trainer experimentally and physically. Our struggles for a complete understanding continue.
References
- [1] D. Aoki, J.-P. Brison, J. Flouquet, K. Ishida, G. Knebel, Y. Tokunaga, and Y. Yanase, J. Phys.: Condens. Matter 34, 243002 (2022).
- [2] S. Ran, I.-L. Liu, Y. S. Eo, D. J. Campbell, P. Neves, W. T. Fuhrman, S. R. Saha, C. Eckberg, H. Kim, J. Paglione, D. Graf, J. Singleton, and N. P. Butch, Nat. Phys. 15, 1250 (2019).
- [3] A. Miyake, Y. Shimizu, Y. J. Sato, D. Li, A. Nakamura, Y. Homma, F. Honda, J. Flouquet, M. Tokunaga, and D. Aoki, J. Phys. Soc. Jpn. 88, 063706 (2019).
- [4] A. Miyake, Y. Shimizu, Y. J. Sato, D. Li, A. Nakamura, Y. Homma, F. Honda, J. Flouquet, M. Tokunaga, and D. Aoki, J. Phys. Soc. Jpn. 90, 103702 (2021).
- [5] S. Imajo, Y. Kohama, A. Miyake, C. Dong, J. Flouquet, K. Kindo, and D. Aoki, J. Phys. Soc. Jpn. 88, 083705 (2019).
- [6] A. Miyake, H. Mitamura, S. Kawachi, K. Kimura, T. Kimura, T. Kihara, M. Tachibana, and M. Tokunaga, Rev. Sci. Instrum. 91, 105103 (2020).
- [7] A. Miyake, M. Gen, A. Ikeda, K. Miyake, Y. Shimizu, Y. J. Sato, D. Li, A. Nakamura, Y. Homma, F. Honda, J. Flouquet, M. Tokunaga, and D. Aoki, J. Phys. Soc. Jpn. 91, 063703 (2022).
- [8] A. Ikeda, Y. H. Matsuda, and H. Tsuda, Rev. Sci. Instrum. 89, 096103 (2018).
- [9] M. Gen, A. Miyake, H. Yagiuchi, Y. Watanabe, A. Ikeda, Y. H. Matsuda, M. Tokunaga, T. Arima, and Y. Tokunaga, Phys. Rev. B 105, 214412 (2022).