Difference in Curvature Sensing of Isotropic and Anisotropic Curvature-Inducing Proteins
Noguchi Group
In living cells, biomembrane shapes are controlled by various curvature-inducing proteins. We have studied the binding of these proteins using mean-field theories. Clathrin and coat protein complexes bend membranes in a laterally isotropic manner and generate spherical buds. In contrast, Bin/Amphiphysin/Rvs (BAR) superfamily proteins bend the membrane along their BAR domain axes and generate cylindrical membrane tubes. The former and latter proteins can be modeled as laterally isotropic objects and anisotropic objects of a crescent elliptic shape, respectively.
For isotropic proteins, we derived the free energy, including the Gaussian curvature and area expansion by the protein insertion [1]. Then, we investigated the formation of spherical buds [1] and binding onto a tethered vesicle [2]. Experimentally, a narrow membrane tube (tether) is elongated from a spherical vesicle by an external force imposed by a micropipette and optical tweezers. A first-order transition occurs between a small number of large buds and a large number of small buds with increasing chemical potential of protein binding [2]. For the tethered vesicle, interestingly, a first-order transition occurs twice between low and high protein densities in the tube. The force-dependence curves of the protein density in the membrane tube and the tube curvature are reflection symmetric and point symmetric, respectively, from the force point, in which the tube curvature matches the protein (sensing) curvature (see Figs. 1(a) and (b)) [2]. This theory reproduces the meshless membrane simulation results of the homogeneous phases very well. In addition, beaded-necklace-like tubes with microphase separation were found in the simulation.
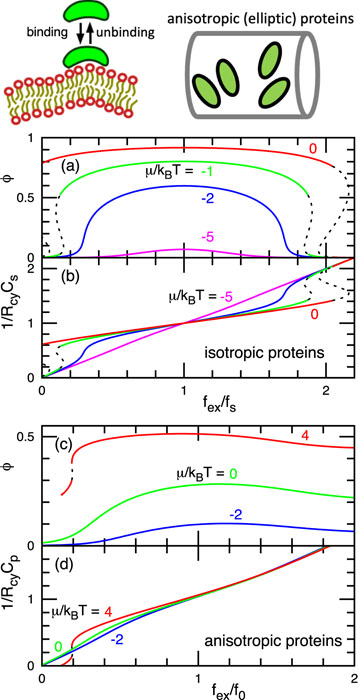
Fig. 1. Binding of proteins onto tubular membranes. External force fex dependence of protein density φ and tube radius Rcy. (a),(b) Isotropic proteins with sensing curvature Cs. (c),(d) Anisotropic proteins with a spontaneous curvature Cp along proteins axis. With increasing binding chemical potential m, more proteins are bound.
For anisotropic proteins, an orientational-dependent excluded volume interaction is included in the free energy. We studied binding onto membrane tubes [3]. The proteins exhibit a second-order or first-order nematic transition with increasing protein density for intermediate and small radii of the membrane tube, respectively. The tube curvatures for the maximum protein binding (sensing) and orientational order are differently varied by the protein density and rigidity. As the external force along the tube axis increases, a first-order transition from a large tube radius with low protein density to a small radius with high density occurs only once, and subsequently, the protein orientation tilts to the tube-axis direction (see Figs. 1 (c) and (d)). The density-dependent sensing curvature and the number of transitions are characteristics due to the anisotropic bending energy. In contrast, each isotropic protein has a constant sensing curvature. This density-dependent sensing curvature has been measured in the experiment of BAR proteins. This theory quantitatively reproduces the results of meshless membrane simulation for short proteins. For long proteins, the formation of protein clusters generates a quantitative deviation from the theory, although qualitative agreements still hold.
The present theories can be used to estimate the bending rigidity and curvature of bound proteins in experiments and atomistic simulations. These mechanical parameters are keys to quantitatively understand the curvature sensing and generation of proteins.
References
- [1] H. Noguchi, Phys. Rev. E 104, 014410 (2021).
- [2] H. Noguchi, Soft Matter 17, 10469 (2021).
- [3] H. Noguchi, C. Tozzi, and M. Arroyo, Soft Matter 18, 3384 (2022).