Higher-Order Topological Insulator Built from van der Waals Stacking of Bismuth-Halide Chains
Kondo Group
A Z2 weak topological insulator (WTI) state can be formed in a material with stacked quantum spin Hall insulators (QSHIs), where the topological surface states appear only on side surfaces by accumulating helical edge states of QSHIs [1]. Similarly, a higher-order topological insulator (HOTI) is expected to be built from stacking QSHIs so that 1D helical hinge states are formed by accumulating the edge states. Utilizing symmetry-based indicators, the HOTI phases have been predicted in materials which were previously regarded as trivial insulators [2]. So far, only bulk bismuth has been experimentally shown to be in the HOTI phase [3]. However, bismuth is a semimetal, whose bulk cannot become insulating by carrier doping. Therefore, the experimental realization of a HOTI in an insulating material has been awaited.
In this study, we propose that quasi-1D bismuth halides offer a novel platform to investigate various topological phases that depend on the stacking sequences of Bi4X4 (where X is I or Br) chains. A WTI state has been observed in β-Bi4I4 with single-layered chains per unit cell, while a normal insulator state has been obtained in α-Bi4I4, which takes a double-layered structure (Fig. 1) [1]. Although Bi4Br4 also consists of double layers, each layer is alternately rotated by 180 degrees, leading to the prediction of a HOTI phase due to the slight shift of the inversion center in the unit cell. Based on this, we present angle-resolved photoemission spectroscopy (ARPES) results showing that Bi4Br4 is a HOTI for the first time as a real 3D material [4].
An advantage of employing quasi-1D materials lies in the formation of a number of steps aligned parallel to the chain direction on the cleavage surface. Figure 2(a) shows a photographic image of ribbon-like Bi4Br4 crystals used in the experiments. While the cleaved surface is relatively rough, as shown in Fig. 2(b), Grazing incidence Small-angle X-ray Scattering (GISAXS) measurements has revealed that the surface consists only of the top (001) surface and the side (100) surface. This result indicates that a huge number of hinges between the two planes are exposed on the cleaved surface, offering the possibility of detecting the topological hinge states by ARPES measurements.
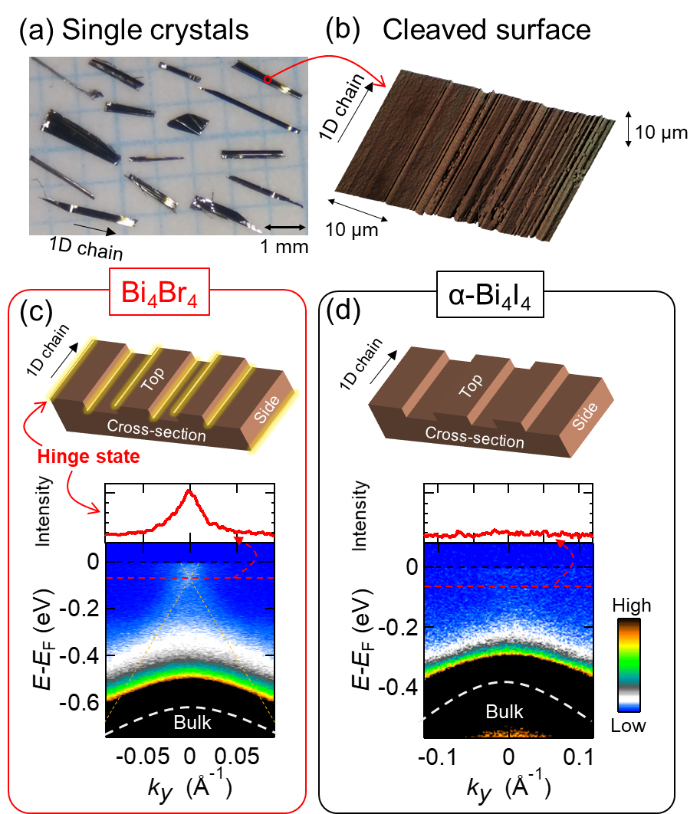
Fig. 2 (a) Photograph of Bi4Br4 crystals. (b) Laser microscope image of a cleaved surface. (c) ARPES band map of Bi4Br4 around the Γ point (bottom) and the corresponding momentum distribution curve at E-EF=-60 meV (middle). Schematic of the cleaved surface of Bi4Br4 with topological hinge states (top). (d) Similar results to (c), but for α-Bi4I4.
Figure 2(c) presents the ARPES result of Bi4Br4 taken by high-resolution laser-ARPES. The strong photoemission signals observed at ~0.6 eV below the Fermi level (EF) are assigned to the bulk valence band. Interestingly, we also observed metallic in-gap states with Dirac-like dispersions around EF (bottom panel of Fig. 2(c)). This is further confirmed in the momentum distribution curves at the energy of the roughly estimated Dirac point (middle panel of Fig. 2(c)). The in-gap states are found to form a quasi-1D intensity distribution in the Fermi surface mapping. These observations are in stark contrast to the case for a trivial insulator α-Bi4I4, where no indication for in-gap states is found in ARPES image or the corresponding momentum distribution curve (Fig. 2(d)). Therefore, the 1D Dirac state in Bi4Br4 is attributed to the topological states, demonstrating the realization of the HOTI phase in Bi4Br4. Our comprehensive study on bismuth halides also shows that the difference in chain stacking is the origin of the different band topologies among Bi4Br4, α-Bi4I4, and β-Bi4I4. The quasi-1D HOTI state we have verified in this study will bring the new capability of designing and engineering functional materials utilizing the stacking-dependence of the topological phases.
References
- [1] R. Noguchi et al., Nature 566, 518 (2019).
- [2] F. Tang, H. C. Po, A. Vishwanath, and X. Wan, Nat. Phys. 15, 470 (2019).
- [3] F. Schindler et al., Nat. Phys. 14, 918 (2018).
- [4] R. Noguchi et al., Nat. Mater. (2021). https://doi.org/10.1038/s41563-020-00871-7