Origin of Inverse Magnetocaloric Effects in Ni-Mn-based Heusler Alloys
T. Kihara, X. Xu, and M. Tokunaga
The development of heat-exchange systems using the magnetocaloric effect is a topic where material science can solve energy problems. The magnetocaloric effect has long been used to realize ultra-low temperature using the adiabatic demagnetization procedure. This effect was recently expected for heat exchangers with higher efficiency than conventional gas-compression/expansion systems [1]. However, most of the materials that exhibit significant magnetocaloric effect contain heavy rare-earth elements, so that the high cost of the materials hinders their practical use. We have studied thermodynamic properties of Heusler alloys without rare-earth elements to realize the giant magnetocaloric effect involving the entropy of the lattice and electronic systems [2-5].
Ni-Mn-based Heusler alloys have attracted considerable attention as magnetic shape memory alloys [6]. In our previous work on Ni-Co-Mn-In alloys, we directly observed prominent negative magnetocaloric effects at the field-induced phase transitions from paramagnetic martensite to ferromagnetic austenite phase. From the systematic high-field studies of magnetocaloric effect, magnetization, heat capacity, and microscopy, we concluded that this effect originated from the increase of lattice entropy associated with the structural phase transition [2].
In the present study, we measured the magnetization, magnetocaloric effect, and magnetostriction of Ni-Co-Mn-Ga alloys in pulsed high magnetic fields up to 56 T [4, 5]. As shown in Fig. 1(a), metamagnetic transitions similar to those in the Ni-Co-Mn-In alloy were observed in this material. Magnetostriction measured on a polycrystalline sample using the capacitance method [7] showed a significant lattice distortion associated with this transition [Fig. 1(b)], suggesting that this magnetic transition is accompanied by a structural phase transition from the martensite to the austenite phase.
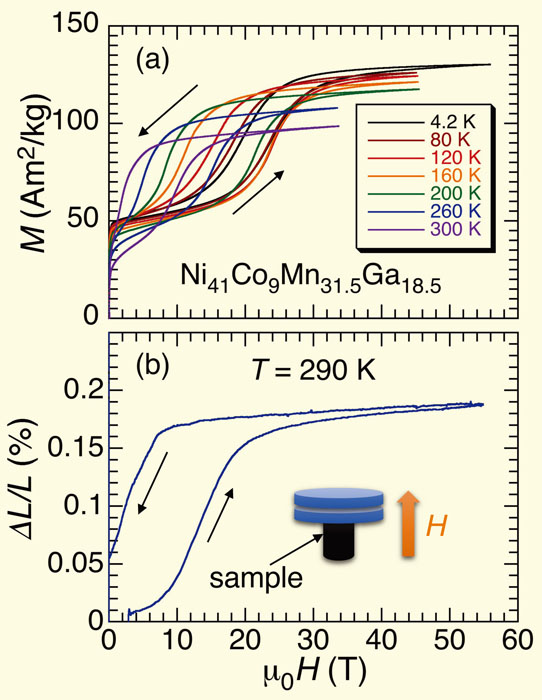
Fig. 1. (a) Field-dependence of magnetization in a polycrystal of Ni41Co9Mn31.5Ga18.5 at several temperatures. Steep increase in magnetization indicates emergence of metamagnetic transitions. (b) Longitudinal magnetostriction of Ni41Co9Mn31.5Ga18.5 measured at 290 K. The inset shows schematics of the capacitance method. Prominent increase of the sample length corresponds to the structural transition from the martensite to the austenite phase simultaneously with the metamagnetic transition.
The change in the sample temperature at the magnetic field-induced phase transition shows a negative magnetocaloric effect, as shown in Fig. 2. In contrast to the Ni-Co-Mn-In alloy, the negative magnetocaloric effect shows up even after completing the field-induced magnetic and structural transition. The experimental results of heat capacity and theoretical results of first-principles calculation show that the electronic specific heat coefficient increases significantly at the transition to the austenite phase: the entropy of the electron system plays an essential role in the magnetocaloric effect of this system. The increase in lattice and electronic entropy associated with the magnetic field-induced phase transition dominates over the decrease in the spin entropy change, which shows up as a negative magnetocaloric effect that decreases the sample temperature in a magnetic field. If we can incorporate these entropy changes in the same sign, we expect magnetocaloric effects beyond the limit of the spin system. Microscopic discussion in the present study will pave the way for this giant magnetocaloric effect.
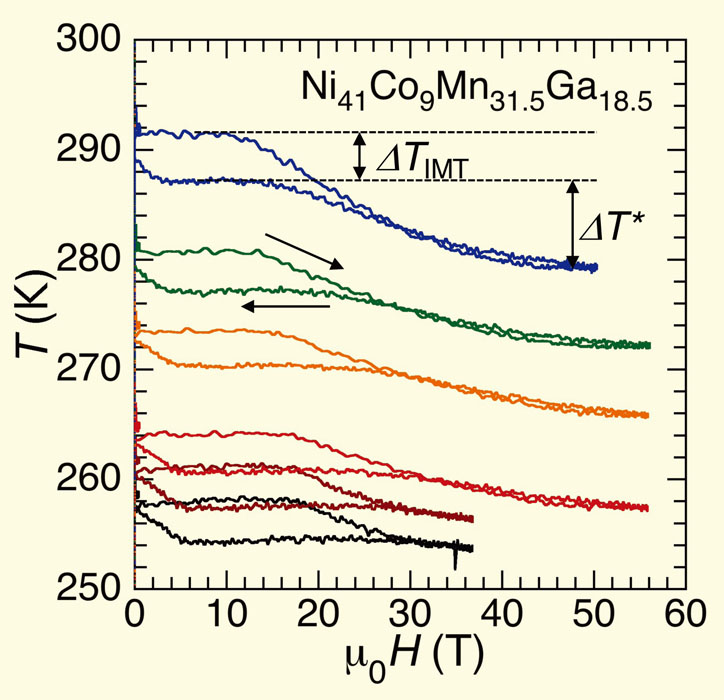
Fig. 2. Magnetocaloric effect of Ni41Co9Mn31.5Ga18.5 studied in adiabatic magnetization process realized in pulsed magnetic fields. Instantaneous change in sample temperature is detected using a thin-film resistive thermometer attached to the sample. In addition to steep reduction of temperature at the metamagnetic transition (ΔTIMT), further negative magnetocaloric effect is observed at higher fields (ΔT*).
Most of the previous studies on magnetocaloric materials have focused on their properties in the magnetic field region below 5 T. In the future society where hydrogen energy and high-temperature superconducting cables are put to practical use. In this situation, large-scale application of the magnetocaloric effects using large superconducting magnets, such as gas liquefaction projects, will also be important. The evaluation of the magnetocaloric effects in Heusler alloys at high magnetic fields provides an essential hint for solving energy problems in near future.
References
- [1] K. A. Gschneidner Jr., V. K. Pecharsky, and A. O. Tsokol, Rep. Prog. Phys. 68, 1479 (2005).
- [2] T. Kihara et al., Phys. Rev. B 90, 214409 (2014).
- [3] X. Xu et al., MATEC Web of Conf. 33, 01004 (2015).
- [4] T. Kihara et al., Scr. Mater. 181, 25 (2020).
- [5] T. Kihara et al., Phys. Rev. Mater. 5, 034416 (2021).
- [6] R. Kainuma et al., Nature 439, 957 (2006).
- [7] A. Miyake et al., Rev. Sci. Instrum. 91, 105103 (2020).