Free-Standing Oxide Thin Film Membranes
R. Takahashi and M. Lippmaa
A fundamental parameter that determines the physical properties of crystalline materials is the lattice parameter, which is generally determined by the chemical composition and the lattice type of a crystal. Options for tuning materials properties or electronic phases by changing the lattice parameters is generally limited to the use of thermal expansion, the application of mechanical pressure, or ionic substitutions — also known as chemical pressure. Thin films offer an additional option of using epitaxial strain for lattice parameter control, since nanometer-scale films grown on single-crystal substrates tend to assume the same in-plane lattice parameter as the substrate material. Even in mechanically hard materials, such as oxides, epitaxial tensile or compressive strains of several percent can be routinely obtained. Larger strain states, however, are difficult to obtain without mechanical failure of a crystal. Likewise, it can be difficult to fabricate entirely strain free thin films. An interesting technique that can be used to apply very high local or long-range strain fields on thin layers is the use of free-standing thin films. In our recent work, we have developed a new oxide thin film lift-off process based on the use of lattice-matched BaO sacrificial layers to grow thin films that can be released from a substrate and transferred to a secondary carrier [1]. The technique can be used to extend the achievable range of mechanical strain in oxide materials and is the basis for constructing novel vibrational energy harvesting devices.
The process of fabricating free-standing BaTiO3 films is illustrated in Fig. 1. A sacrificial BaO layer is first grown on a SrTiO3 substrate. The BaO layer is nearly lattice matched with titanate lattices and can be grown epitaxially on the substrate surface. The membrane material, in this case BaTiO3, is grown on top of the sacrificial BaO layer. The heterostructure is then removed from the thin film growth chamber and attached with an adhesive layer to a flexible secondary carrier, such as a glass sheet or even a polymer backing. When the whole structure is immersed in water, the BaO layer dissolves, detaching the single-crystal substrate from the membrane layer, as illustrated in Fig. 1c, which shows the detached membrane on a secondary flexible carrier and the detached substrate. In principle, the substrate can be recycled for growing another thin film.
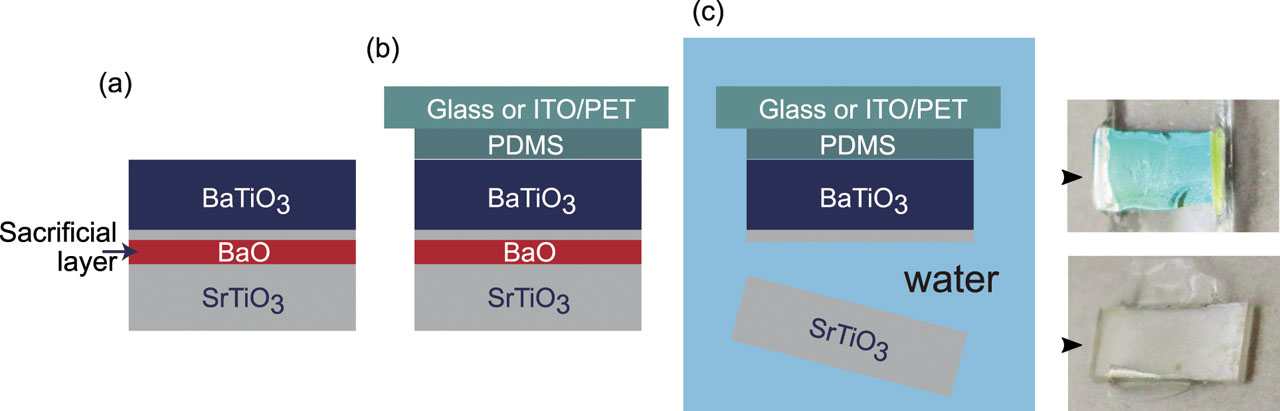
Fig. 1. Free-standing oxide thin film membrane fabrication process: (a) growth of a sacrificial BaO layer and the functional membrane layer, (b) attaching the heterostructure with a poly-dimethylpolysiloxane (PDMS) adhesion layer to a indium tin oxide-coated polyethylene terephthalate (PET) carrier, and (c) removing the substrate by dissolving the BaO layer in water.
One interesting application of the membrane process is the development of piezoelectric energy converters for harvesting vibrational energy. The use of a membrane device is benefitial because the piezoelectric film, such as BaTiO3, can be grown epitaxially under optimal growth conditions on a high-quality single crystal substrate, yielding the highest piezoelectric coefficient. However, the bulk substrate prevents the application of large strains on the film, as is required for low-frequency vibrational energy harvesting. Much higher strains can be achieved in a membrane device where the piezoelectric film is detached from the substrate and sandwiched between flexible conducting electrodes, as illustrated in Fig. 2a. An energy harvester device can be constructed by supported a flexible membrane cantilever from one end and attaching a test mass on the free end. When mechanical vibration is applied, a periodic current can be generated in an external circuit, powering, for example, a low-power environmental sensor device. The output signal of a test cantilever using a BaTiO3 film is shown in Fig.2b.
The demonstration of the process used a BaTiO3 film, but the technique itself is applicable to any material that can be grown epitaxially on the sacrificial BaO layer. For energy harvesting devices, a flexible backing is generally required to maintain the integrity of a millimeter-scale membrane.
An obvious use of the technique is to fabricate films that are truly strain free and for this reason, the technique has much broader potential applications than flexible piezoelectric energy conversion devices.
References
- [1] R. Takahashi and M. Lippmaa, ACS Appl. Mater. Interfaces 12, 25042 (2020).