Thermal Hall Effects of Spins and Phonons in Kagome Antiferromagnet Cd-Kapellasite
Yamashita, Hiroi, Kawashima, and Sakakibara Groups
The trajectory of an electron bends as it moves through a magnetic field. This Hall effect has been thought to not appear in insulators because of the apparent absence of mobile electrons. But recent reports of a thermal version of this effect, known as the thermal Hall effect, in numerous insulators has led to broad attention from researchers to understand its origin as well as potential applications for thermal current control.
Thermal current in an insulator is carried by spins and phonons. This leads us to ask how these charge neutral carriers can be bent by magnetic fields and how one can separate these two effects. To clarify the issue, we investigate the magnetic insulator Cd-kapellasite CdCu3(OH)6(NO3)2, a kagomé antiferromagnet [1]. In Cd-kapellasite, Cu2+ ions form a kagomé structure with a dominant nearest-neighbor antiferromagnetic interaction J/kB = 45 K. The geometrical frustration effect of the kagomé structure suppresses the magnetic order down to TN = 4 K, realizing a spin liquid state in a wide temperature range TN < T < J/kB.
We find clear thermal Hall signals in the spin liquid phase in all samples. The thermal Hall conductivity (κxy) is larger in a higher-quality sample with a larger longitudinal thermal conductivity (κxx), whereas the temperature dependence of κxy is similar (Fig. 1(a)). At 15 T, κxy and κxx are found to show a peak at almost the same temperature, a telltale sign of a phonon contribution κ
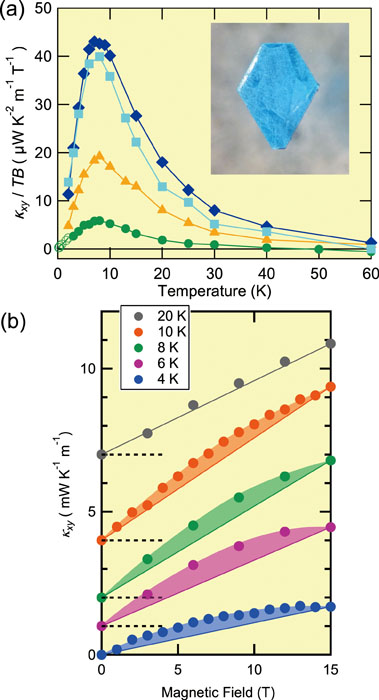
Fig. 1. (a) The temperature dependence of the thermal Hall conductivity of Cd-kapellasite of different samples at 15 T [1]. All the samples show a peak at the same temperature, but with different magnitudes. (b) The field dependence of κxy at different temperatures. The non-linear part (colored region) shows the spin contribution.
Remarkably, by assembling the κxx dependence of κ
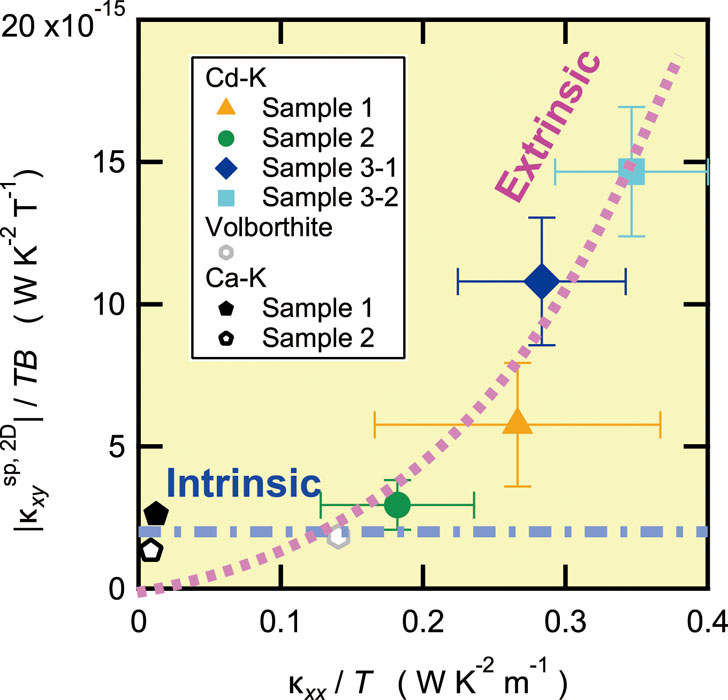
Fig. 2. The dependence of the spin thermal Hall conductivity per the 2D kagomé layer (κ
Furthermore, we find that both κ
References
- [1] M. Akazawa et al., Phys. Rev. X 10, 041059 (2020).
- [2] D. Watanabe et al., Proc. Natl. Acad. Sci. USA 113, 8653 (2016).
- [3] H. Doki, M. Akazawa, H.-Y. Lee et al., Phys. Rev. Lett. 121, 097203 (2018).