Capacitive Detection of Physical Quantities in Pulsed Magnetic Fields
Tokunaga Group
Capacitance is known as a physical quantity that can be measured with high precision. We have developed a system to precisely measure various physical quantities that change in pulsed high magnetic fields using high-speed capacitance measurements [1].
First, we measured magnetostriction. We attach a pair of electrode plates on top of a sample and on the probe with keeping a small gap between them. We can detect small change in the sample length through the change in the capacitance of this setup. Using this method, we observed magnetostriction in a Heusler alloy caused by a significant magneto-volume effect [2]. The entropy change associated with this magneto-volume effect is regarded as the origin of the giant inverse magneto-caloric effect observed in this class of materials [2,3]. Therefore, quantitative evaluation of the structural change can be essential to understand the magnetocaloric effect. In non-magnetic metals, magnetostriction can be used to detect the field-induced changes in band filling. Utilizing this technique, we achieved thermodynamic evidence of complete valley polarization in elemental bismuth in the ultra-quantum limit state [4].
Capacitance measurements are also valuable for studying multiferroic materials in which magnetic order correlates with the dielectric property. If the change in magnetic symmetry makes the crystal polar, we can detect it through electric polarization measurements. On the other hand, the change is hardly discernible in the polarization if the spin order does not break global inversion symmetry, such as in the antiferroelectric state. Our capacitive measurement of dielectric constants enabled to detect this kind of change in symmetry at high magnetic fields. Figures 1 show the magnetization and dielectric constant of single crystals of Pb(TiO)Cu4(PO4)4. In contrast to the broad hump structure in the magnetization curve, dielectric constant shows a steep change around 26 T with field hysteresis, which unambiguously indicates the change in magnetic symmetry at this field. This technique provides us with a novel tool to identify the symmetry of magnetic phases emerging in high magnetic fields.
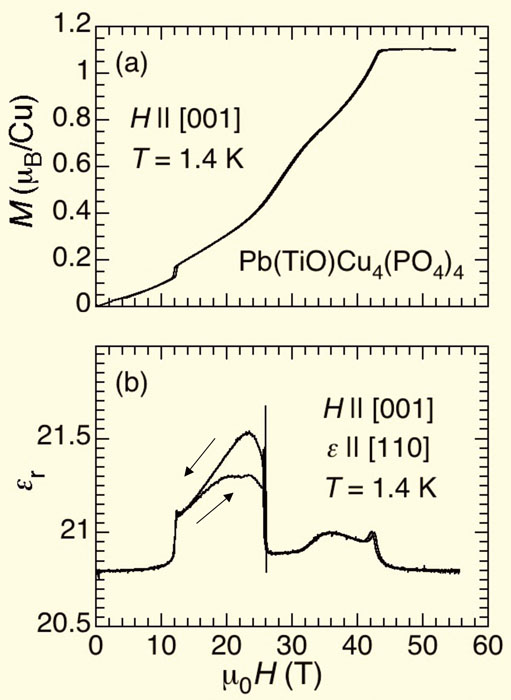
Fig. 1. Field dependence of (a) magnetization and (b) real part of the relative dielectric constant of Pb(TiO)Cu4(PO4)4. Successive transitions of cluster spin multipole order show up as multiple anomalies in both physical quantities. Broad hump structure in magnetization around 26 T becomes prominent in the dielectric constant measured using the capacitance method.
By measuring dielectric constants of some non-magnetic materials attached to a sample, we can evaluate the temperature change of a sample in magnetic fields: the so-called magneto-caloric effect. We show an example of the measurements of the magneto-caloric effect using KTa1-xNbxO3 (x = 0.02) as a capacitance thermometer, whose dielectric constant has a temperature dependence as shown in Fig. 2(a). We have previously developed a similar system to measure the magneto-caloric effect using a thin-film resistance thermometer [5]. Although this method works effectively, we have to calibrate the effect of magnetoresistance in the thermometer in advance at each temperature. On the other hand, in the present capacitance thermometer, the capacitance is almost insensitive to the applied magnetic field, as shown in Fig. 2(b). Using this thermometer, we can successfully measure the magneto-caloric effect of a magnetic refrigerant Gd3Ga5O12 in a pulsed magnetic field (Fig. 2(c)). Due to the non-magnetic nature of this thermometer, we can also measure magnetocaloric effects simultaneously with magnetization. Such simultaneous measurements will enable us to precisely determine the field-temperature phase diagram of all the kind of magnetic materials and to discuss more precise thermodynamics.
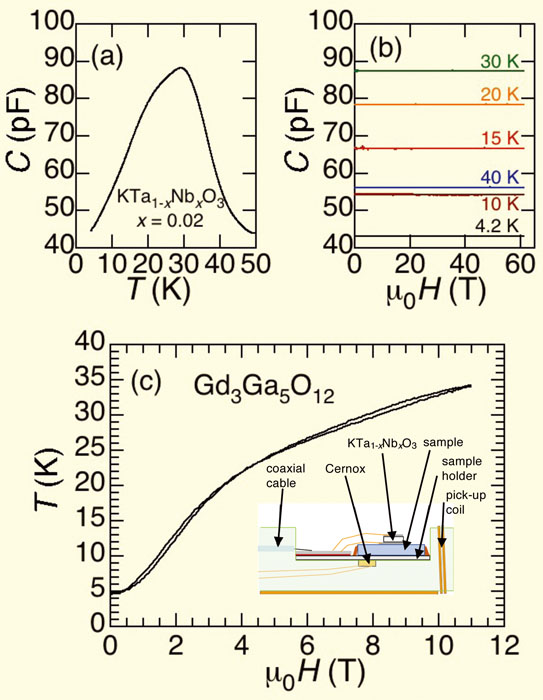
Fig. 2. (a) Temperature variation of the capacitance of KTa1-xNbxO3 at zero magnetic field. (b) Magnetic field dependence of the capacitance of KTa1-xNbxO3 at various temperatures. These two results indicate that this material can be used as a capacitance thermometer insensitive to a magnetic field. (c) Magnetocaloric effects in Gd3Ga5O12 measured using this capacitance thermometer. The inset shows schematics of the experimental setup. Our fast capacitance measurements enable to evaluate the magnetocaloric effect in this material in a pulsed magnetic field.
High precision measurements of capacitance improve evaluation of the structure, symmetry, and entropy changes of the sample in pulsed magnetic fields. We expect that such a technological innovation will open up new horizons in science at high magnetic fields.
References
- [1] A. Miyake et al., Rev. Sci. Instrum. 91, 105103 (2020).
- [2] T. Kihara et al., Phys. Rev. Mater. 5, 034416 (2021).
- [3] T. Kihara et al., Phys. Rev. B 90, 214409 (2014).
- [4] A. Iwasa et al., Sci. Rep. 9, 1672 (2019).
- [5] T. Kihara et al., Rev. Sci. Instrum. 84, 074901 (2013).