Low Gilbert Damping in Epitaxial Thin Films of the Nodal-Line Semimetal D03-Fe3Ga
Miwa and Nakatsuji Groups
D03-ordered Fe3Ga (Fig. 1(a)) is a nodal-line semimetal and shows a giant anomalous Nernst effect (ANE). The origin of the ANE was attributed to the large density of states (DOS) and the large Berry curvature near the Fermi level. The large Berry curvature emerges as a result of spin-orbit-coupling-induced gap opening along the nodal lines consisting of nearly flat valence and conduction bands touching each other. This peculiar band structure may be useful for spintronics device application because a gate voltage may effectively modulate Berry-curvature-associated responses as well as magnetic anisotropy. In this regard, it is important to characterize the magnetization dynamics of D03-Fe3Ga. For such a purpose, we fabricated epitaxial thin films of D03-Fe3Ga and bcc-Fe and performed ferromagnetic resonance (FMR) measurements to evaluate their Gilbert damping constants.
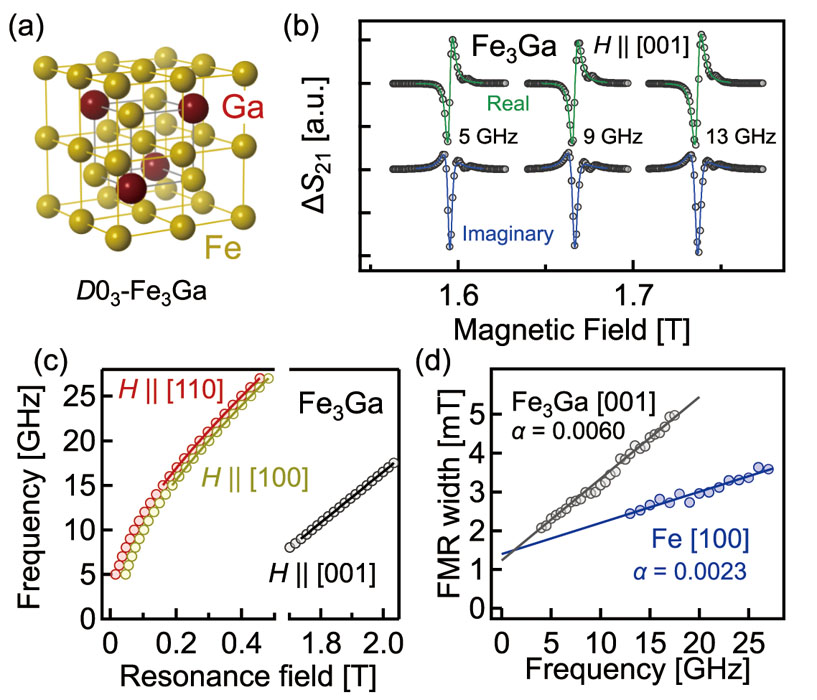
Fig. 1. (a) Crystal structure of D03-Fe3Ga. (b) Typical Ferromagnetic resonance (FMR) spectra of D03-Fe3Ga. (c) Resonance frequency plotted against resonance magnetic fields. The solid curves are the fits using the Kittel formula. (d) FMR peak widths of D03-Fe3Ga and bcc-Fe. The solid lines represent the linear fits, the slope of which represents the intrinsic Gilbert damping constant.
50-nm-thick D03-Fe3Ga and Fe thin films were grown by dc magnetron sputtering and molecular beam epitaxy, respectively. We confirmed that the D03-Fe3Ga layer was grown epitaxially by performing high-energy electron diffraction and x-ray diffraction measurements. We conducted FMR measurements using a vector network analyzer and a coplanar waveguide. We employed a field-modulation technique [2], which measures the magnetic-field (H) derivative of the transmission coefficient ΔS21, to minimize field-independent background signals.
Figure 1(b) shows typical FMR spectra of the Fe3Ga thin film taken with magnetic fields applied along the [001] direction. Here, a FMR spectrum refers to ΔS21 as a function of magnetic field at a fixed frequency. From the FMR spectra, we deduced the peak positions and peak widths, which are shown in Figs. 1(c) and 1(d), respectively. We fitted the Kittel formula to the FMR peak positions, and the fits are shown by solid curves in Fig. 1(c). We found that the in-plane cubic anisotropy H4// of Fe3Ga favors the magnetization along the [110] direction (H4// ~ −15 mT), while those of Fe and disordered Fe-Ga alloys favor the [100] direction (H4// ~ + 60 mT for Fe and H4// ~ + 75-100 mT for the Fe-Ga alloys [3]). This magnetic-anisotropy change indicates that the D03 atomic ordering significantly alters the electronic band structure of Fe-Ga alloys and induces the giant ANE as a result.
The FMR peak widths of the Fe and Fe3Ga thin films [Fig. 1(d)] showed linear behavior, the slope of which corresponds to the Gilbert damping constant. The linear fits yielded the Gilbert damping constants of α = 0.0023 ± 0.0002 for Fe and α = 0.0060 ± 0.0002 for D03-Fe3Ga. These values are somewhat consistent with what is expected from the theoretical DOSs, indicating that Ga atoms do not significantly increase the spin-orbit coupling strength. Although the Gilbert damping constant of D03-Fe3Ga is larger than that of bcc-Fe, it is still low compared to typical ferromagnets such as CoFeB (α ~ 0.004-0.006) [4] and Permalloy (α ~ 0.007) [5]. In other words, the nodal lines near the Fermi level do not hinder the magnetization dynamics much. The low Gilbert damping may be useful for spintronics device applications because one may be able to efficiently manipulate the magnetization direction by exploiting the peculiar band structure of D03-Fe3Ga.
In summary, we have investigated the magnetization dynamics of the epitaxial thin films of the nodal-line semimetal D03-Fe3Ga by ferromagnetic resonance measurements. We have deduced the intrinsic Gilbert damping constant of D03-Fe3Ga to be (6.0 ± 0.2) × 10−3, which is larger than the Fe Gilbert damping constant of (2.3 ± 0.2) × 10−3 but as low as other typical ferromagnets. The low Gilbert damping and the peculiar band structure with a large Berry curvature make D03-Fe3Ga attractive as a building block of spintronics devices.
References
- [1] A. Sakai, S. Minami, T. Koretsune, T. Chen, T. Higo, Y. Wang, T. Nomoto, M. Hirayama, S.Miwa, D. Nishio-Hamane, F. Ishii, R. Arita, and S. Nakatsuji, Nature 581, 53 (2020).
- [2] S. Tamaru, S. Tsunegi, H. Kubota, and S. Yuasa, Rev. Sci. Instrum. 89, 053901 (2018).
- [3] S. Budhathoki, A. Sapkota, K. M. Law, B. Nepal, S. Ranjit, S. KC, T. Mewes, and A. J. Hauser, J. Magn. Magn. Mater. 496, 165906 (2020).
- [4] A. Conca, J. Greser, T. Sebastian, S. Klingler, B. Obry, B. Leven, and B. Hillebrands, J. Appl. Phys. 113, 213909 (2013).
- [5] Y. Zhao, Q. Song, S.-H. Yang, T. Su, W. Yuan, S. S. P. Parkin, J. Shi, and W. Han, Sci. Rep. 6, 22890 (2016).