Giant Effective Damping of Octupole Oscillation in a Chiral Antiferromagnet Mn3Sn
Miwa, Nakatsuji, and Otani Groups
Understanding of spin dynamics forms the basis of spintronic application. For the ultrafast THz control of staggered moments in antiferromagnets, the time-resolved observation of spin dynamics is essential for developing the associated technology. However, such observation has been limited to insulators to date. Recently, the metallic anttiferromagnets Mn3X have attracted significant attention for its strong response (e.g. anomalous Hall effect) comparable to ferromagnets owing to the hidden ferroic order, which configures large Berry curvature originated from Weyl points in a momentum space. Such ferroic order can be characterized by cluster magnetic octupoles based on neighbouring magnetic moments, and thus it is highly important to clarify the dynamics of cluster octupole for designing the spintronic application. In this research, we conduct time-resolved observation of spin-dynamics in a chiral antiferromagnet Mn3Sn.
Bulk single-crystal D019-Mn3Sn has been employed. Mn3Sn has the hexagonal Ni3Sn-type crystal structure consisting of an ABAB stacking of the kagome lattice of Mn atoms along the [0001] axis. Red (blue) circles in Fig. 1 indicate Mn atoms in A- (B-) plane of the kagome lattice. An inverse triangular spin structure is stabilized by exchange and Dzyaloshinskii-Moriya interactions [1,2]. The inverse triangular spin structure is made of a ferroic ordering of cluster magnetic octupoles [3].
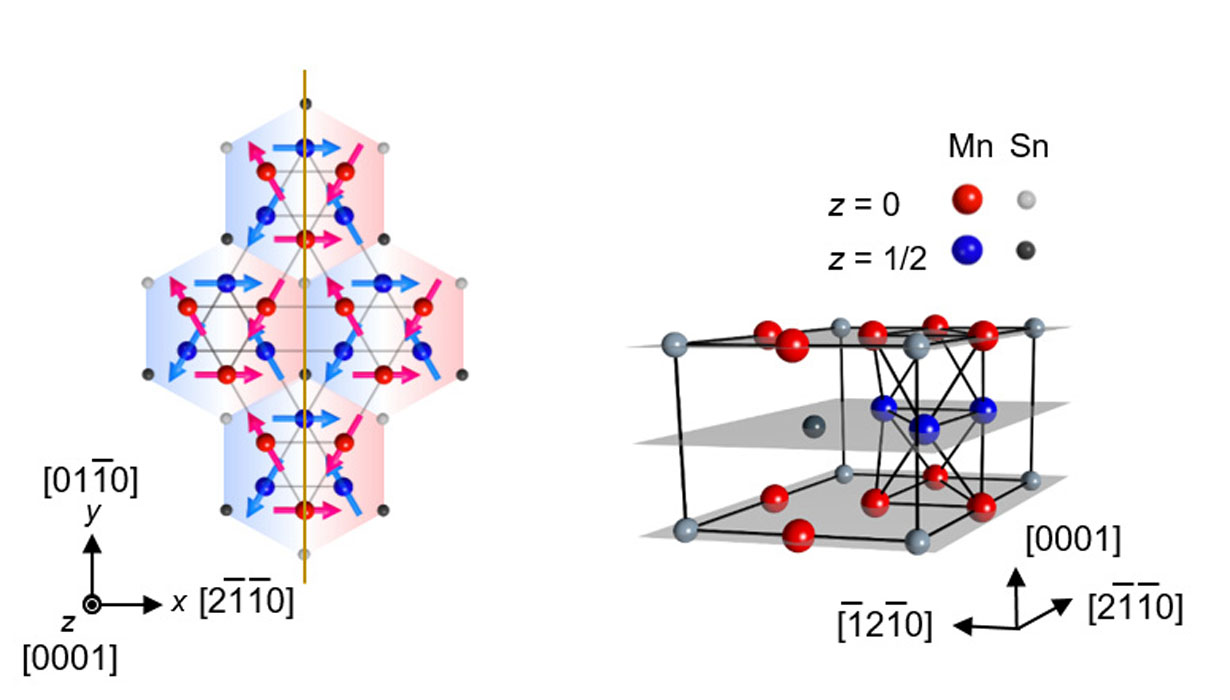
Fig. 1. (a) Spin and crystal structures of Mn3Sn. Different colors are used to denote Mn and Sn atoms in the z = 0 and z = 1/2 planes. The inverse triangular spin-structure can be viewed as a ferroic ordering of cluster magnetic octupole, which possesses the same symmetry as the spin-magnetization (e.g. as indicated by a mirror plane (orange line)).
Figure 2(a) shows typical TR-MOKE results, where a magnetic field of 2 T was applied normal to the surface. First, a pump light induces rapid decrease in the polar MOKE intensity of a probe light, similar to ultrafast demagnetization observed in ferromagnetic metals [4]. Consecutively, the MOKE intensity start to recover with delay time more than 0.3 ps, exhibiting a small but clear oscillation during the recovery. To further characterize the oscillating component, a non-oscillating component was estimated as a background (black solid curve) and subtracted from the raw data. The lower panel of Fig. 1(b) shows the TR-MOKE signals after subtracting the background. Figure 1(c) also shows typical TR-MOKE results (upper panel) and the analysis for the oscillating component (lower panel) in a relatively long-time range. From the fits in the lower panel of Figs. 1(b) (orange curve) and 1(c) (blue curve), oscillation frequencies (ωI, ωII) are determined as a function of an external magnetic field, namely, ωI = 0.86 THz and ωII = 18 GHz. The oscillating behaviour is most significant when the magnetic field is normal to the Mn3Sn surface. Because TR-MOKE signal is proportional to the surface normal polarization of the cluster magnetic octupoles, the oscillating Kerr signal should come from a change of size of the cluster magntic octupole order.
Two oscillation modes I and II are found through the analyses shown in Figs. 1(b) and 1(c), which should come from the dynamics of the cluster magnetic octupoles. The mode I and II are assigned to the optical and collective-precession-like modes. From Fig. 1(c), we find that the dynamics of the cluster octupole in Mn3Sn is enhanced by exchange interaction, and it is feasible to conduct an ultrafast switching at < 10 ps, a hundred times faster than the case of spin-magnetization in a ferromagnet. Moreover, our theoretical analysis with the aforementioned experimental results shows that the Néel type domain wall velocity in Mn3Sn can be estimated as > 10 km/s, which is greater than the recently reported value (~2 km/s) for the ferrimagnetic systems in the vicinity of the angular momentum compensation temperature [5]. Significantly, our study provides the first demonstration of the time-resolved observation of the spin-wave oscillation in an antiferromagnetic metal.
References
- [1] S. Nakatsuji, N. Kiyohara, and T. Higo, Nature 527, 212 (2015).
- [2] S. Tomiyoshi and Y. Yamaguchi, J. Phys. Soc. Jpn. 51, 2478 (1982).
- [3] M.-T. Suzuki, T. Koretsune, and M. Ochi, Phys. Rev. B 95, 094406 (2017).
- [4] E. Beauprepaire et al., Phys. Rev. Lett. 76, 4250 (1996).
- [5] K.-J. Kim et al., Nat. Mater. 16, 1187 (2017).