High-Magnetic-Field X-Ray Diffraction Study in Solid Oxygen
Y. Matsuda Group
Molecular oxygen is a unique homonuclear diatomic molecule because it possesses a spin quantum number S = 1. After M. Faraday had noticed that gaseous oxygen exhibits magnetism, condensed phases, namely liquid and solid phases have been extensively studied as intriguing magnetic materials. Solid oxygen undergoes successive phase transitions with lowering temperature, and three distinct phases, γ-phase, β-phase, and α-phase appear at 54, 44, and 24 K, respectively. One of the most intriguing findings on these three phases is that they have different crystal symmetries, meaning that the successive phase transitions are not merely magnetic phase transitions but structural phase transitions. The γ-phase, β-phase, and α-phase have a cubic, rhombohedral, and monoclinic crystal structure, respectively. The lowest temperature phase, the α-phase is an antiferromagnet at a low temperature below 24 K, while the β-phase only exhibits a short-range antiferromagnetic correlation and the γ-phase is paramagnetic. We have been sharing the idea that magnetic energy between oxygen molecules should play an important role for having different crystal structure for a long time. However, no direct experimental evidence of the strong spin-lattice coupling had ever been obtained before the discovery of the novel magnetic-field-induced θ-phase at 120 T [1]. It has been proved that controlling spins induces a novel oxygen crystal.
Microscopic investigation of the crystal lattice of solid oxygen in magnetic fields is important. Actually, there was a report of the high magnetic field x-ray diffraction of the solid oxygen using a synchrotron x-rays up to 8 T and significantly large magnetostriction was likely to occur in the α-phase of solid oxygen [2]. The volume striction ΔV/V was found to reach around 10-2. In the present study, we have extended the magnetic field range to 25 T for the x-ray diffraction experiment.
The high-field XRD experiments have been performed at the Institute for Materials Research of Tohoku University with a DC magnet up to 5 T, and at BL22XU in SPring- 8 up to 25 T using a pulsed magnet. Pure oxygen gas (purity > 99.99995%) was liquified by cooling into a sample cell designed for each experiment. For the 5 T DC-magnet experiment, a CuKα (1.54 Å) characteristic emission line was used as the x-ray source. The measurement temperature was controlled to be 10 K. In the 25 T pulse-magnet experiments, the photon energy (wavelength) of the synchrotron x-ray used is 15 keV (0.8266 Å). The time interval of the successive pulsed magnetic field measurements is 10–20 min, which is necessary for cooling the magnet after heating due to the discharge for field generation. The variation of the magnetic field ΔB/B during the measurement time (100 μs) is around 2% [3].
The measured XRD profiles in pulsed magnetic fields at 10 and 25 T are shown in Fig. 1 along with the zero-field profile. The XRD at 0 T is measured with an exposure time of 10 ms. The profiles at 10 and 25 T are obtained by averages of six times and five times the repetition of the measurements, respectively. The obtained XRD profiles at different magnetic fields seem to be identical at first glance.
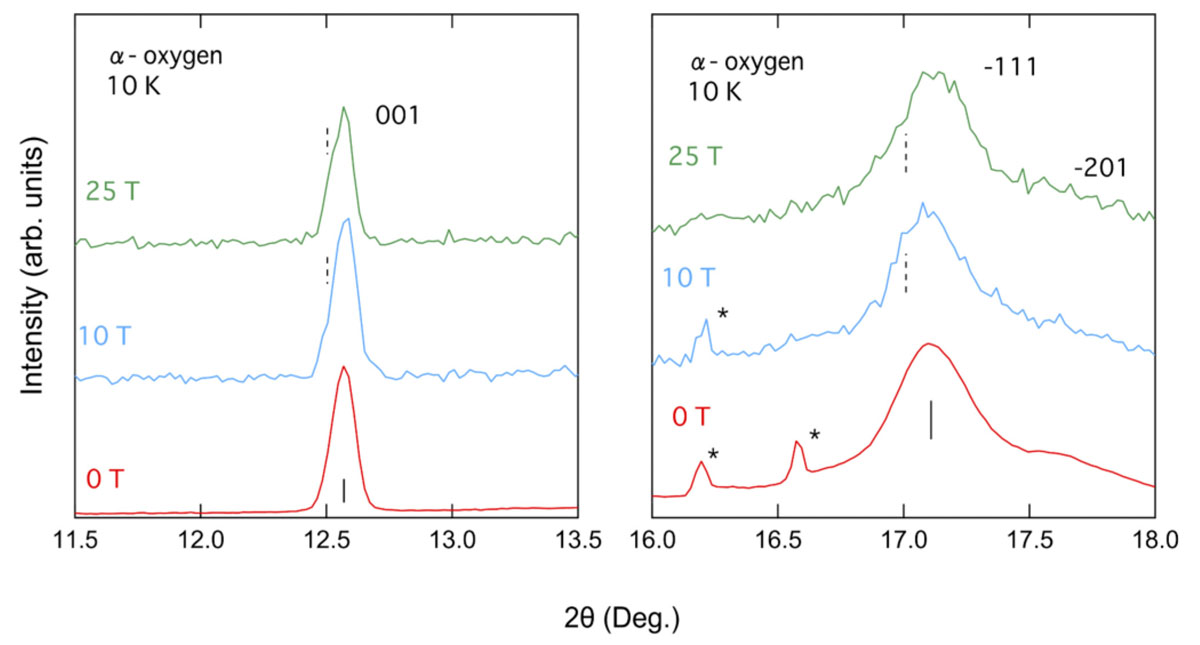
Fig. 1. High-magnetic-field X-ray diffraction profiles of the α-phase of solid oxygen at 10 K. Vertical dashed lines indicate the positions that are expected from the previous report [2]. (a) 001 reflection peaks are shown. (b) -111 and -201 peaks are shown. Peaks with * are considered to be due to impurities on the window of the sample cell.
In order to confirm the unexpectedly small magnetic field effect, we have also analyzed the steady field XRD data that have higher precision in terms of the longer accumulation time. And also, we need to exclude a possibility that the dynamics of the crustal lattice is slower than the pulsed magnetic field of which duration time is 1 ms in this study.
Figure 2 shows the obtained linear magnetostriction Δd/d at 5 T. The Δd/d of several diffraction plane spacing is found to be as small as 10-4 which is more than one order of magnitude smaller than the values reported previously [2].
The reason for the contradiction between our results and the previous study is not very clear. One of the possible causes for the contradiction is that there had been some mechanical influence on the experimental configuration by applying DC magnetic fields. Small mechanical movements of measurement apparatuses can cause a change in the observed XRD peak positions [3].
The high-field XRD experiments on α oxygen at 10 K has revealed that the linear magnetostriction Δd/d is smaller than 10−4 T−1 in magnetic fields of up to 25 T [3]. The absence of the giant magnetostriction in α oxygen is also in agreement with the experimental fact that the magnetic field induced α-θ transition is first order [1]. The deformation of the crystal lattice in a magnetic field is not significant below the critical magnetic field around 100 T. It is likely that a drastic symmetry change in the crystal lattice takes place discontinuously at the phase transition.
References
- [1] T. Nomura, Y. H. Matsuda, S. Takeyama, A. Matsuo, K. Kindo, J. L. Her, and T. C. Kobayashi, Phys. Rev. Lett. 112, 247201 (2014).
- [2] K. Katsumata, S. Kimura, U. Staub, Y. Narumi, Y. Tanaka, S. Shimomura, T. Nakamura, S. W. Lovesey, T. Ishikawa, and H. Kitamura, J. Phys.: Condens. Matter 17, L235 (2005).
- [3] Y. H. Matsuda, A. Shimizu, A. Ikeda, T. Nomura, T. Yajima, T. Inami, K. Takahashi, T. C. Kobayashi, Phys. Rev. B 100, 214105 (2019).