Strain-Induced Magnetic Weyl Semimetal in an Epitaxial Thin Film of a Luttinger Semimetal
Nakatsuji, Lippmaa, Katsumoto, and Kindo Groups
Strong electronic correlations serve as a central thread of highly unusual behaviors in quantum materials, ranging from metal-insulator transitions to unconventional superconductivity. The interplay of correlated electron physics with recently discovered novel topological phases establishes a new, fascinating research direction that awaits a full exploration. A vital example of this kind is the magnetic Weyl semimetal (WSM), in which the strongly enhanced Berry curvature inherent to the linearly dispersing crossing points between two electronic bands (i.e., the Weyl points) allows for the emergence of surprisingly large anomalous transport effects even in antiferromagnets or spin-liquid candidates. While the WSM is earlier identified experimentally in nonmagnetic materials with breaking inversion symmetry [1,2], the search for magnetic WSM states in strongly correlated systems remains challenging yet of great importance, due to their excellent tunability and appealing potential for innovative technological applications.
The earliest predicted magnetic WSM is the family of pyrochlore iridates R2Ir2O7 (where R is a lanthanoid or yttrium) [3], a 5d electron system featuring noncoplanar spin configurations in both 5d and 4f electron sectors. The 5d series provides an ideal ground for realizing exotic topological phases, owing to the comparable strength of the spin-orbit interaction relative to the Coulomb repulsion, allowing the correlation physics to play a critical role. Indeed, recent experiments identified Pr2Ir2O7, the metallic end-member of the R2Ir2O7 family, as a Luttinger semimetal comprising quadratic band touching of doubly degenerate valence and conduction bands at the Brillouin zone center Γ near the Fermi level EF (Fig. 1a) [4]. Lattice strain or magnetic field tuning of the Luttinger semimetal may engender a rich topological phase diagram, and is considered a promising route towards realization of a magnetic WSM [5,6].
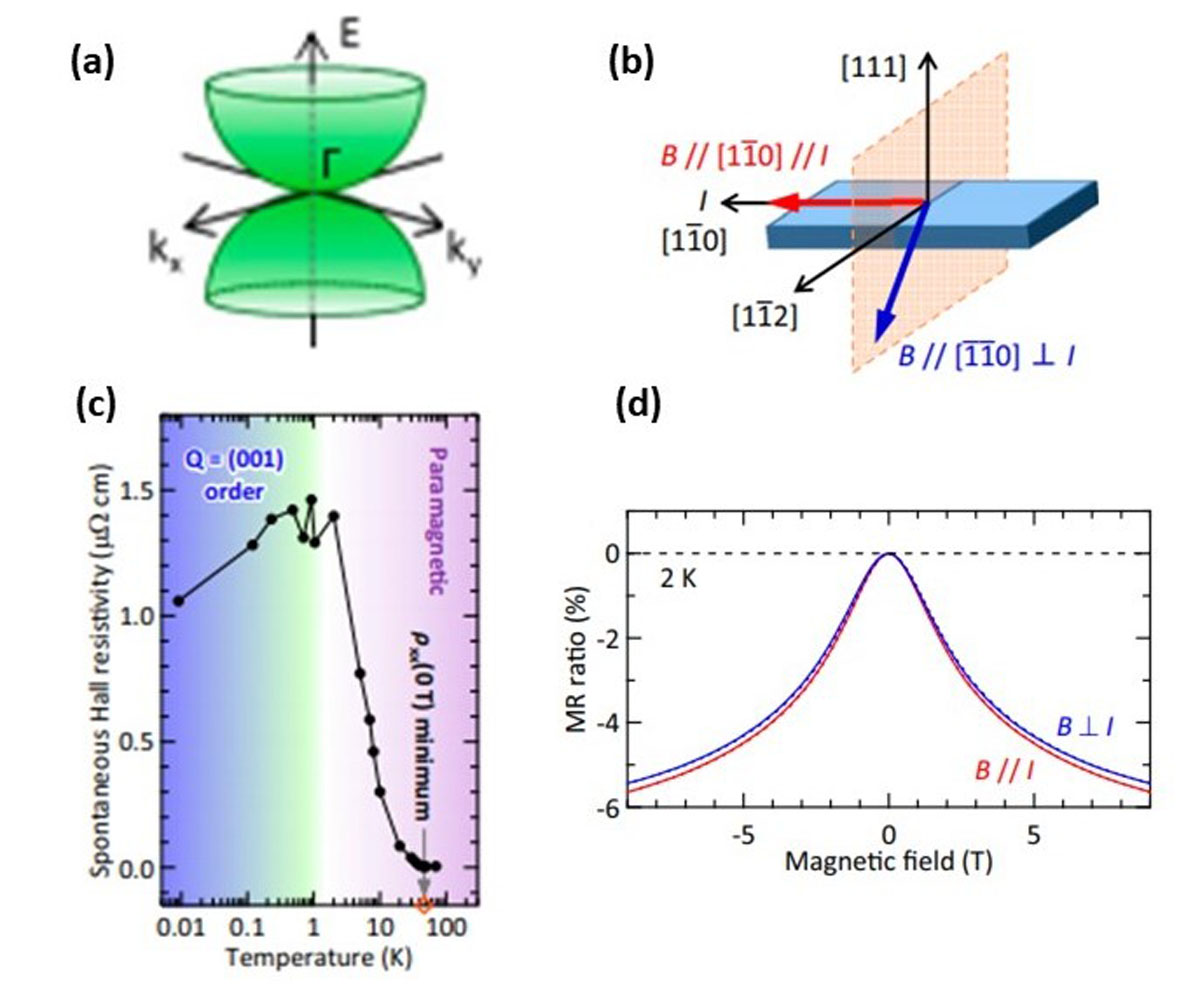
Fig. 1. (a) Band structure of a Luttinger semimetal consisting of quadratic band touching of 5d electrons at the Γ point near EF (b) Schematic for experimental configurations of magnetotransport measurements. (c) Temperature dependence of the spontaneous Hall resistivity. (d) Magnetoresistance curves as a function of the magnetic field B measured at 2 K for B || I and B ⊥ I configurations. The solid and dotted lines are up and down sweeps of B, respectively. The difference between the two curves arises from the chiral anomaly and indicates that the film is in the Weyl semimetal state.
Recent studies on Pr2Ir2O7 have focused on its bulk properties, yet the difficulties of applying uniaxial strain on bulk single crystals leave the magnetic WSM shrouded in mystery. In contrast, thin films allow for control over the crystal growth orientation and lattice deformation via compressive or tensile strain imposed by epitaxial lattice mismatch with a substrate. Here, we report the transport properties of our epitaxial Pr2Ir2O7 thin films, which provide firm evidence for a magnetic WSM state induced by the tensile strain along the surface normal [111] direction [7].
Using strained Pr2Ir2O7 thin films, we observed a zero-field Hall effect persisting up to about 50K without detectable spontaneous magnetization (Fig.1c). This onset temperature of the spontaneous Hall effect is much higher than the value (~ 1.5K) observed in bulk single crystals and is nearly two orders of magnitude higher than the exchange coupling (J ~ 0.7K) of Pr 4f moments [8], indicating the breaking of time-reversal symmetry induced by the magnetic order of Ir 5d electrons. Thus, the condition for realizing a magnetic WSM state is fulfilled in the strained thin film. Moreover, we identified a negative contribution to the magnetoresistance specific to the chiral anomaly (Fig. 1 b and d) and the planar Hall effect (Fig. 2); both phenomena are considered the fingerprints of Weyl points lying close to EF [9, 10, 11].
In summary, we succeeded in synthesizing epitaxial thin films of Pr2Ir2O7 and revealed key transport signatures of the WSM under strain tuning. The discovery of strain-induced magnetic Weyl semimetal state in pyrochlore iridates thin films paves a new avenue to explore topological phases in strongly correlated materials.
References
- [1] B. Q. Lv et al., Phys. Rev. X 5, 031013 (2015).
- [2] S.-Y. Xu et al., Science 349, 613 (2015).
- [3] X. Wan et al., Phys. Rev. B 83, 205101 (2011).
- [4] B. Cheng, T. Ohtsuki et al., Nat. Commun. 8, 2097 (2017).
- [5] E.-G. Moon et al., Phys. Rev. Lett. 111, 206401 (2013).
- [6] T. Kondo et al., Nat. Commun. 6, 10042 (2015).
- [7] T. Ohtsuki et al., Proc. Natl. Acad. Sci. U.S.A. 116, 8803 (2019).
- [8] Y. Machida et al., Nature 463, 210 (2010).
- [9] D. T. Son and B. Z. Spivak, Phys. Rev. B 88, 104412 (2013).
- [10] A. A. Burkov, Phys. Rev. B 96, 041110(R) (2017).
- [11] S. Nandy et al., Phys. Rev. Lett. 119, 176804 (2017).