Electrical Manipulation of a Topological Antiferromagnetic State
Nakatsuji, Otani, and Miwa Groups
Recently, materials with nontrivial band topology have attracted considerable attention due to their novel physical properties. One such example is a Weyl semimetal, in which two non-degenerate bands linearly touch in momentum space, forming gapless Weyl fermions with different chiralities in a time-reversal-symmetry (TRS) or inversion-symmetry breaking state. These touching points or Weyl nodes act as topologically protected, unit-strength monopoles or antimonopoles of underlying Berry curvature such as a large anomalous Hall effect (AHE) and chiral anomaly. The electrical manipulation of these novel properties becomes an important topic for developing new technologies utilizing novel topological state. For this purpose, TRS-breaking or magnetic Weyl semimetals would be suitable owing to their magnetic texture. Moreover, given the prospects of antiferromagnetic (AF) spintronics for realizing high-density devices with ultrafast operation [1], electrical manipulation of an AF Weyl metal is ideal, but have not yet been reported. In this study, we demonstrate the electrical switching of a topological AF state and its detection by AHE at room temperature in a Weyl antiferromagnet Mn3Sn by using spin-orbit torque (SOT) switching method.
Mn3Sn has a hexagonal D019 structure with the ABAB-stacking sequence of a (0001)-kagome layer of Mn, and the geometrical frustration leads to a three-sublattice non-collinear AF ordering of Mn spins below the Néel temperature TN ~ 430 K (Fig. 1(a)) [2]. The magnetic octupole’s polarization direction determines the location of Weyl nodes and the associated distribution of the Berry curvature in the momentum space (Fig. 1(b) right). To induce possible SOT switching in Mn3Sn, we prepared the Mn3Sn/nonmagnetic metal (NM = Pt, W, Cu) bilayer devices. Figure 2(a) shows the schematic image of the SOT switching in Mn3Sn/Pt bilayer devices. When an electrical current flows into the Pt layer, a spin polarized current generated by the spin Hall effect of Pt induces the SOT on the Mn3Sn layer and causes the switching of the polarization direction of the magnetic octupole. The measurement configuration and optical micrograph of the fabricated bilayer Hall bar devices are shown in Fig. 2(b). In our study, we first perform the magnetic field switching of the Hall voltage VH by using an out-of-plane magnetic field Hz with a read current of 0.2 mA. A clear hysteresis of VH with the zero-field change of ΔVHfield (= VH(+Hz→0) − VH(−Hz→0)) is observed (Fig. 2(c)). To examine the possible SOT switching of Mn3Sn, we pass a 100 ms write-pulse current Iwrite along the x-direction; then, we wait for 600 ms after the pulse current and measure VH with a 0.2 mA read current. Fig. 2(d) shows VH as a function of Iwrite in Pt, W, and Cu devices. For the NM = Pt (spin Hall angle θSH > 0), a clear negative (positive) jump appeared in the Hall voltage under a positive (negative) current larger than a critical threshold write-current Ic with a bias field Hx along the current direction x. The magnitude of the jump reaches ~30 % of the total Hall voltage change |ΔVHfield | in the field sweep measurements. To confirm that this switching of VH is related to the SOT from the spin Hall effect in the NM layer, we prepared NM = W (θSH < 0) and Cu (θSH ~ 0) instead of Pt. For NM = W, the switching polarity is opposite to the Pt one, while for NM = Cu, there is almost no hysteresis with the electrical current cycle. The difference in switching polarity between Pt and W devices and the absence of switching in Cu devices cannot be accounted for by the Oersted field generated by the electrical current but agree well with the sign of θSH of the NM layer. These results demonstrate that SOT from the NM layer induces the perpendicular switching of the AF domain and thus manipulates the direction of Weyl nodes.
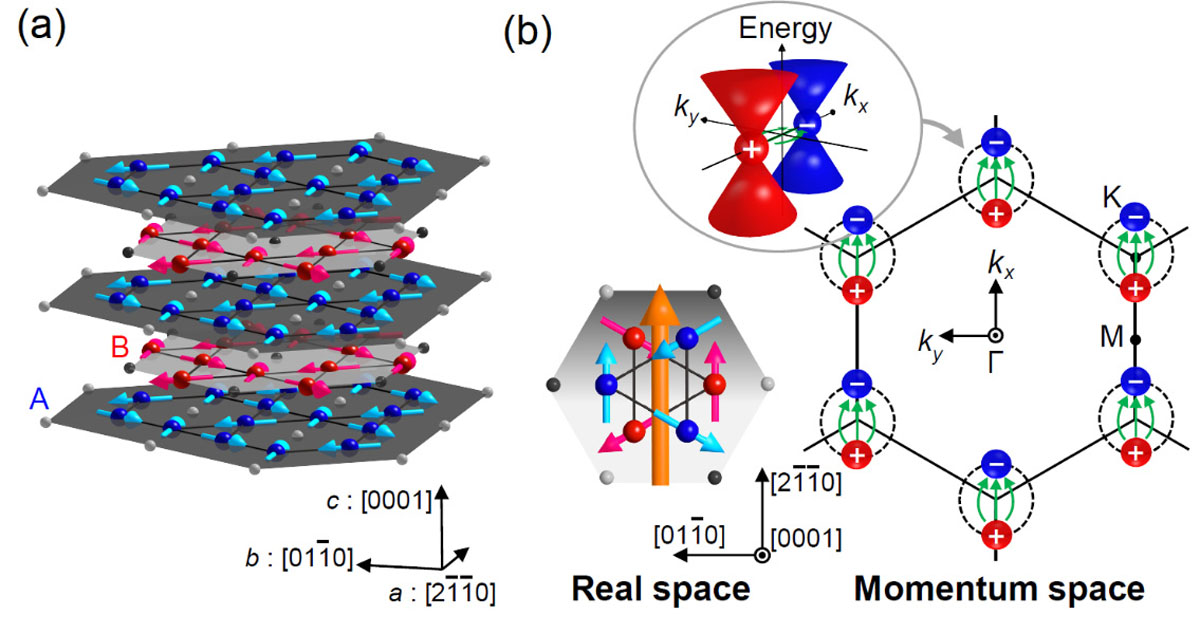
Fig. 1. (a) Mn3Sn crystal structure and inverse triangular spin (ITS) structure. The large blue and red spheres (small gray and black spheres) represent Mn atoms (Sn atoms) at z = 0 and 1/2, respectively. The Mn magnetic moments (light blue and pink cylindrical arrows) lie within the kagome-layer with the AB-AB stacking sequence and form the ITS structure at room temperature. The spin structure on the kagome bilayers can be viewed as a ferroic ordering of a cluster magnetic octupole. (b) Left: cluster magnetic octupole (orange cylindrical arrow) consisting of the six spins on the kagome bilayer. Right: schematic distribution of the Weyl points near the Fermi energy in the momentum space (kx-ky plane at kz = 0) for the magnetic structure shown in the left-side figure. Red and blue spheres correspond to Weyl nodes, which respectively act as sources (+) and drains (-) of the Berry curvature (green arrows). Inset: three-dimensional schematic picture of a pair of Weyl nodes.
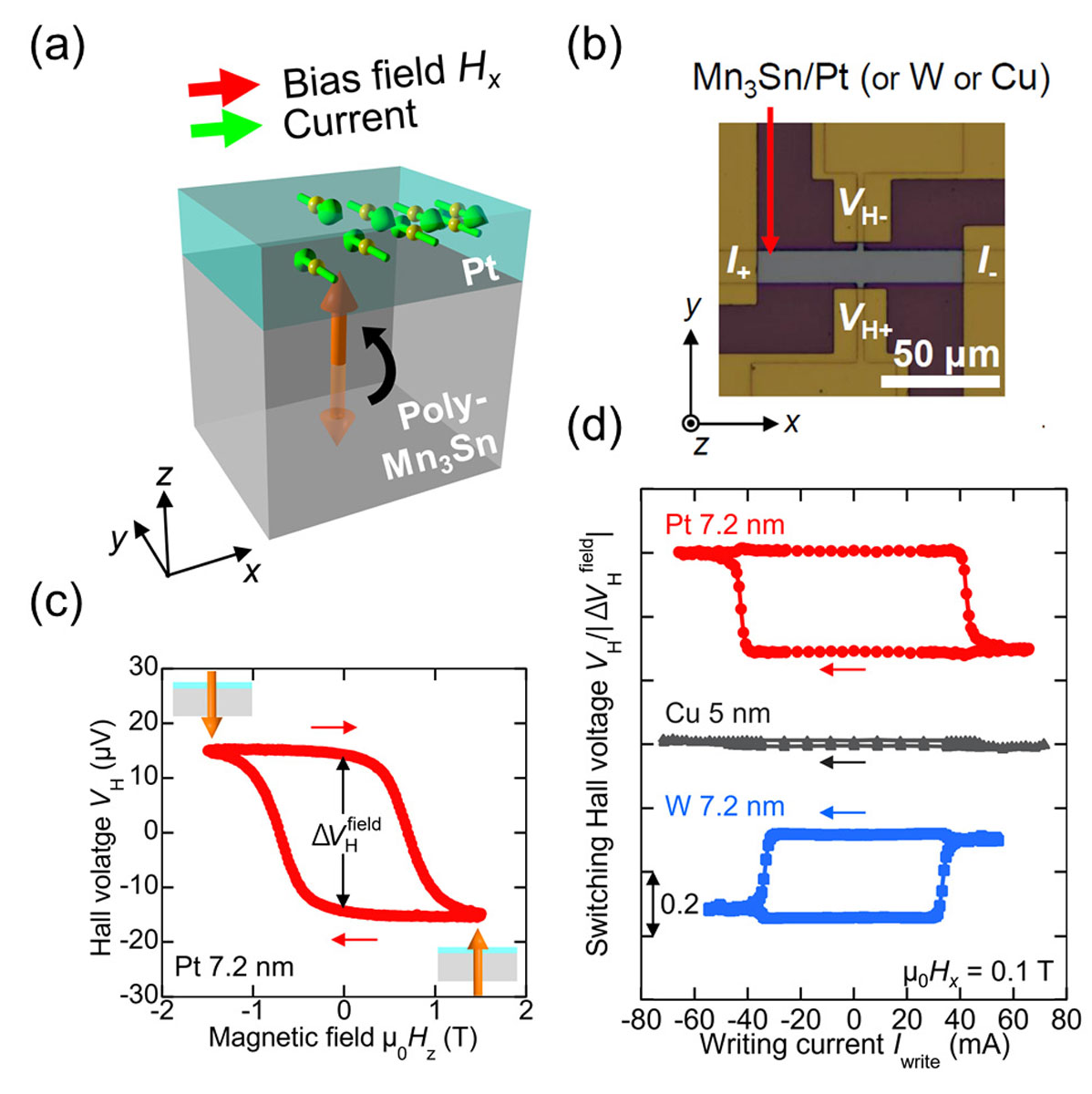
Fig. 2. (a) Schematic image for the spin-orbit torque switching. The spin-polarized current (green cylindrical arrows on yellow spheres) generated in Pt exerts a spin-orbit torque, causing the switching of the polarization axis of the cluster magnetic octupole (orange cylindrical arrow) in the polycrystalline Mn3Sn under a write current and a bias field along the x-direction. (b) Measurement configuration and optical micrograph of the fabricated Mn3Sn-nonmagnet bilayer Hall bar devices. (c) Hall voltage VH vs. magnetic field along the z-direction Hz for the Mn3Sn/Pt 7.2 nm device at room temperature. Inset: Schematic figures illustrating the direction of the cluster magnetic octupole. (d) Hall voltage VH vs. write current Iwrite for the Pt 7.2 nm, Cu 5 nm, and W 7.2 nm devices at room temperature. The Hall voltage is normalized by the zero-field Hall voltage |ΔVHfield| in the magnetic field dependence for each sample.
In recent years, utilizing antiferromagnets in spintronics devices have attracted significant attention as its vanishing small stray fields and much faster spin dynamics than ferromagnets [1]. Our electrical switching measurement of AF Weyl metals is made by using the same protocol as the one used for ferromagnetic metals [3,4]. Moreover, the switching or critical write current density Jc in the NM layer is found reasonably small. It is estimated to be 2 × 1011 A/m2 for 7.2 nm thick Pt and 5 × 1010 A/m2 for 7.2 nm thick W devices, which are smaller than the original values reported for the first observations of the electrical switching in the NM/FM devices (Jc ~ 1012 A/m2) [3,4]. These results indicate that topological antiferromagnets may replace ferromagnets in spintronics devices.
In summary, we demonstrated the electrical switching of Weyl antiferromagnets Mn3Sn by using Mn3Sn/NM bilayer devices. Our findings indicate that SOT switching is a useful tool for electrically manipulating the distribution of Weyl points and Berry curvature in momentum space. These observations may lead to further scientific and technological advances in topological magnetism and antiferromagnetic spintronics [5].
References
- [1] T. Jungwirth et al., Nat. Nanotechnol. 11, 231 (2016).
- [2] S. Nakatsuji, N. Kiyohara, and T. Higo. Nature 527, 212 (2015).
- [3] I. M. Miron et al., Nature 476, 189 (2011).
- [4] L. Liu et al., Science 336, 555 (2012).
- [5] H. Tsai, T. Higo, K. Kondou, T. Nomoto, A. Sakai, A. Kobayashi, T. Nakano, K. Yakushiji, R. Arita, S. Miwa, Y. Otani, and S. Nakatsuji, Nature 580, 608 (2020).