Lithium-Ion Conductivity in Single Crystals of Li10GeP2S12
Hiroi Group
The all-solid-state lithium-ion battery has been studied extensively as a next-generation secondary battery. It uses a solid instead of a liquid electrolyte, which enables to achieve greater safety, higher energy density, wider temperature range of operation, and larger output power compared with the conventional battery. To realize this, an efficient Li-ion conductor is required, which exhibits conductivity that is either comparable to or larger than that of commercially used organic liquid electrolytes. Li10GeP2S12 (LGPS) is a promising candidate for the super ionic conductor; compared to other candidates, it has the highest conductivity of 12 mS cm–1 at room temperature and an outstanding electrochemical performance in Li batteries [1,2]. Thus, LGPS is a key compound in the materialization of the all-solid-state battery.
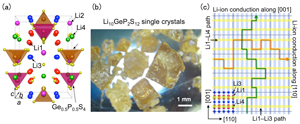
Fig. 1. (a) Crystal structure of Li10GeP2S12 (LGPS), (b) photograph of typical LGPS crystals of a few millimeters in size, and (c) schematic drawing illustrating the possible conducting paths of Li ions in LGPS. The one-dimensional channel via the Li1–Li3 path along the c axis is dominant, and a two-dimensional hopping via the Li1–Li4 path in the <110> direction is secondary in the short-range Li-ion conduction. However, the actual long-range conduction occurs via such meandering paths as shown by the green and orange lines in (c), which give rise to weakly anisotropic Li-ion conductivity.
The high Li-ion conductivity of LGPS has been ascribed to its specific crystal structure shown in Fig. 1a. There are four partially occupied Li sites inside the rigid framework composed of the tetrahedral units of Ge0.5P0.5S4 and PS4. Among them, the Li1, Li3, and Li4 sites are located in the distorted tetrahedra of S, while the Li2 site is in the distorted octahedron of S. It has been shown that the Li diffusion between the former sites is crucial for the high conductivity, while the Li2 ions are almost immobile.
A highly anisotropic ion diffusion has been proposed based on neutron diffraction (ND) measurements and molecular dynamics calculations: a one-dimensional (1D) channel via the Li1–Li3 path along the c axis is dominant, and a two-dimensional (2D) hopping via the Li1–Li4 path in the <110> direction is secondary [3]. The activation energies of the local hopping processes for the 1D and 2D paths are determined as 0.16 (0.17) and 0.26 (0.28) eV from the NMR experiments (the molecular dynamics calculations), respectively. Thus, considerable anisotropy can be expected in the atomic-scale diffusion process. In contrast, pulsed-field gradient NMR measurements, which may be sensitive to long-range Li diffusion at the micrometer scale, suggests a nearly isotropic Li diffusion.
An impedance measurement using a single crystal provides important information with which to clarify the origin of this difference and the mechanism of ion diffusion in LGPS. However, because of the lack of a sufficiently large single crystal, the Li diffusion in the bulk had not been measured thus far. Previous impedance measurements using polycrystalline samples yielded an average conductivity of ~10 mS cm–1 and activation energy of 0.22–0.28 eV. Understanding of the true conduction mechanism in LGPS is crucial for the improvement of LGPS and for further development of a better solid electrolyte for the all-solid-state battery. In the present study, we obtain large single crystals of LGPS, a few millimeters in size, using the self-flux method (Fig. 1b), and carry out impedance measurements in the [001] and [110] directions [4]. The conductivity is, in fact, higher along [001] than along [110]; however, the difference is only a factor of 4. In addition, the activation energies are nearly equal for the two directions, suggesting a weak anisotropic 3D diffusion in LGPS, as schematically illustrated in Fig. 1c.
References
- [1] N. Kamaya, K. Homma, Y. Yamakawa et al., Nat. Mater. 10, 682 (2011).
- [2] Y. Kato, S. Hori, T. Saito et al., Nat. Energy 1, 16030 (2016).
- [3] X. Liang, L. Wang, Y. Jiang et al., Chem. Mater. 27, 5503 (2015).
- [4] R. Iwasaki, S. Hori, R. Kanno, T. Yajima, D. Hirai, Y. Kato, and Z. Hiroi, Chem. Mater. 31, 3694 (2019).