A Linear Correlation between Photocatalytic Activity and Photoexcited Carrier Lifetime
K. Ozawa, S. Yamamoto, and I. Matsuda
Laser-assisted time-resolved (TR) measurement techniques have facilitated great advances in our understanding of excited charge carrier dynamics. Preceding TR experiments have revealed many aspects of photocatalytic properties of TiO2. One of them is that a higher photocatalytic activity of anatase TiO2 (a-TiO2) than rutile TiO2(r-TiO2) results from a longer lifetime of the photoexcited carriers, owing to the different bulk band gap type (direct versus indirect types). On the other hand, it has also been recognized that the TiO2 photocatalytic activity depends on the orientation of the crystal surfaces. Although several mechanisms such as a surface-dependent redox potential, anisotropic diffusion of the carriers, electron-trapping probability, etc. have been proposed as a possible origin, none of them comprehensively explains the surface dependence. Thus, a more detailed picture of the relation between the carrier dynamics and the photocatalytic activity must be drawn to understand the unique phenomenon.
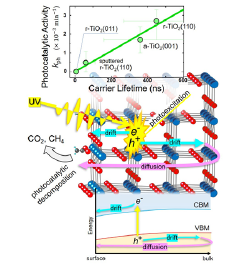
Fig. 1. Correlation between the photocatalytic activity and the carrier lifetime (top). Schematic illustrations of the photoexcited carrier dynamics in a real space (middle) and an energy space (bottom).
In the present study, we systematically assessed the photocatalytic activities and photoexcited carrier lifetime on single-crystal a-TiO2(101) and (001) surfaces and r-TiO2(110), (001), (011), and (100) surfaces by X-ray photoelectron spectroscopy (XPS) and TR-XPS [1]. The photocatalytic activity was evaluated by a rate constant of a photocatalytic decomposition of acetic acid. The carrier lifetime was determined from a time evaluation of the ultraviolet (UV)-induced surface photovoltage (SPV).
Acetic acid adsorbs dissociatively on the TiO2 surfaces via bonding interaction between the O atoms of carboxylate and the surface Ti atoms. Saturation coverages depend on the surface orientation, but it ranges from 0.41 monolayers [on r-TiO2(011)] to 0.66 monolayers [on r-TiO2(100)]. The rate of the photocatalytic decompostion of acetic acid was estimated from a decreasing rate of the C 1s peak intensity while the UV laser with the energy of 3.31 eV was irradiated. The peak intensity decreases exponentially with a time constant τph of 370 min on r-TiO2(110). Since the reciprocal of τph is a rate constant kph, kph on r-TiO2(110) is evaluated to be 2.7×10-3 min-1. This is the largest rete constant among all surfaces examined in the present study. kph on a-TiO2(101) and (001) are 2.1×10-3 min-1 and 1.7×10-3 min-1, respectively, while r-TiO2(011) and (001) are inactive with kph = 0 min-1.
The lifetime of the photoexcited carriers was determined from the time evolution of the SPV-induced Ti 2p peak shift. All TiO2 surfaces examined in the present study form an accumulation layer at the surface because of the inevitable formation of surface oxygen vacancies. The SPV is generated on these surfaces by charge separation of the photexcited electron-hole pairs as illustrated in Fig. 1 (middle and bottom); the electrons and the holes are drifted towards the surface and bulk sides, respectively, along the potential gradient in the accumulation layer. The holes, then, diffuse back to the surface and recombine with the electrons at the surface. This charge separation-recombination process is the origin of the SPV generation-decay process. By examining the SPV decay time after the swift generation by TRXPS, the photoexcited carrier lifetime can be estimated. We examined the carrier lifetimes on three pristine surfaces [r-TiO2(110), r-TiO2(011), and a-TiO2(001)] and one defective r-TiO2(110) surface [2]. It is known that the lifetime strongly depends on the surface barrier height. Thus, the experimentally determined lifetimes were converted to barrier-height-corrected lifetimes so as to reproduce the lifetimes on the acetate-saturated surfaces; they are 450 ns, 360 ns, and 7 ns for r-TiO2(110), a-TiO2(001), and r-TiO2(011), respectively.
The lifetime is found to be proportional to kph. A plot of kph against the lifetime gives a linear and positive correlation (top of Fig. 1). The linear correlation is extendable even to the sputtered surface [1]. On the other hand, no such correlation is found between kph and the surface chemical reactivity, which should be related to the saturation coverage of acetic acid. The photocatalytic reaction is initiated by an interaction between adsorbates and photoexcited carriers at the surface. The present study indicates that the carrier density on the surface rather than the adsorbate density is a prime factor to determine the photocatalytic activity, since more carriers are available for the reaction when the carriers survive longer. Thus, the carrier lifetime is a key to understand various properties of TiO2 photocatalysis, including the orientation-dependent activity.
References
- [1] K. Ozawa, S. Yamamoto, R. Yukawa, R.-Y. Liu, N. Terashima, Y. Natsui, H. Kato, K. Mase, and I. Matsuda, J. Chem. Phys. C 122, 9562 (2018).
- [2] K. Ozawa, S. Yamamoto, R. Yukawa, R. Liu, M. Emori, K. Inoue, T. Higuchi, H. Sakama, K. Mase, and I. Matsuda, J. Chem. Phys. C 120, 29283 (2016).