Spin Current Noise of the Spin Seebeck Effect and Spin Pumping
M. Matsuo and T. Kato
In the research field of mesoscopic physics, current noise measurement is an important tool to obtain useful information on electronic transport such as determination of the effective charge, evaluation of electron entanglement, and even spin accumulation. In the research field of spintronics, the pure spin current induced by, e.g., spin pumping and spin Seebeck effect (see the upper two schematic pictures in Fig. 1), is a central research subject. Recently, the noise of this pure spin current has measured by using the inverse spin Hall effect [1]. Although the noise of the pure spin current is expected to have useful information, its theoretical study has, however, been overlooked for a long time, and has just started recently in a few papers [2].
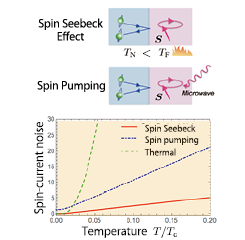
Fig. 1. Upper two pictures: Schematics of spin-current generation by spin Seebeck effect and spin pumping. Lower panel: Temperature dependence of spin-current noise. The temperature is normalized with the Curie temperature of the ferromagnetic insulator. Temperature dependence of thermal spin-current noise is also shown.
In our study [3], we considered a normal metal(NM)/ferromagnetic insulator(FI) bilayer system, which is an important platform of spintronics. We considered a general model Hamiltonian with both spin-conserving and non-spin-conserving processes, and formulated the spin current and the spin-current noise within the framework of Keldysh Green’s function [4]. Using the second-order perturbation with respect to the NM-FI interface coupling, we derived expressions of them in terms of the propagators of electrons and magnons. The lower panel of Fig. 1 shows temperature dependence of the spin-current noise using parameters for a yttrium-iron-garnet-(YIG-)platinum interface. As indicated from the figure, temperature dependences of the spin-current noise contain useful information, which can distinguish the mechanism of the spin-current generation.
We also demonstrated that simultaneous measurement of the spin current and the non-equilibrium spin-current noise provides important information on spin transport. The Fano factor, which is the ratio between the spin-current noise and the spin current, was shown to be constant at low temperatures for both spin Seebeck effect and spin pumping. This low-temperature Fano factor includes information of effective spin in spin transport, which is ħ if the spin conserving process is dominant. As the weight of the spin non-conserving process increases, the effective spin increases by mixture of the two types of spin transport process. In actual experiments of spin pumping, the spin current can be generated also by spin Seebeck effect due to sample heating by microwave irradiation. Discrimination of spin pumping signal from heating effect has been a problem for long time. We proposed a method to estimate an increase of the sample temperature by combining the spin current and the spin-current noise. Finally, we discussed a spin Hall angle, which indicates an efficiency of the inverse spin Hall effect: conversion of spin current into charge current in metals. In a special situation, we can know the Fano factor a priori. For example, the Fano factor is ħ at low temperatures if the spin non-conserving process can be neglected. For such a situation, we can use it as a standard for the spin current and the spin current noise. By comparing this Fano factor with actual measurement of charge current and current noises in the inverse spin Hall effect, we can obtain the spin Hall angle at the interface.
We hope that the present calculation serves as a bridge between two well-established research areas, mesoscopic physics and spintronic physics. This collaborated research started from close discussion with M. Matsuo who was a visiting professor of ISSP in academic year 2016.
References
- [1] A. Kamra et al., Phys. Rev. B, 90, 214419 (2014).
- [2] A. Kamra and W. Belzig, Phys. Rev. Lett. 116, 146601 (2016); S. Takei and M. Mohseni, Phys. Rev. B 97, 014427 (2018).
- [3] M. Matsuo, Y. Ohnuma, T. Kato, and S. Maekawa, Phys. Rev. Lett. 120, 037201 (2018).
- [4] Y. Ohnuma, M. Matsuo, and S. Maekawa, Phys. Rev. B 96, 134412 (2017).