Search for Topological States in an Organic Dirac Semimetal
Osada Group
A layered organic conductor, α-(BEDT-TTF)2I3, has been known as a two-dimensional (2D) gapless Dirac fermion system, namely, Dirac semimetal, with a pair of spin-degenerated Dirac cones. Generally, the Dirac semimetal is a starting point to obtain various topological insulating phases. In graphene, for example, Haldane showed that the Chern insulator (quantum anomalous Hall insulator) appears by attaching the alternating flux pattern breaking time reversal symmetry (TRS) [1]. On the other hand, Kane and Mele showed that the Z2 topological insulator (quantum spin Hall insulator) appears by introducing the spin-orbit interaction (SOI) [2]. We have experimentally and theoretically explored the topological phases starting from the organic Dirac semimetal.
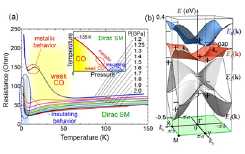
Fig. 1. (a) Temperature dependence of resistance in α-(BEDT-TTF)2I3 at several pressures. (b) Band dispersion of α-(BEDT-TTF)2I3. The symbols, "+" and "–", indicate parity at the time reversal invariant wave numbers.
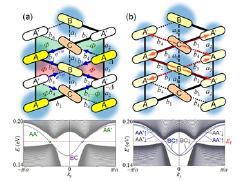
Fig. 2. (a) Flux configuration in the organic Haldane model. The lower panel shows the chiral edge state in the energy spectrum of the nanoribbon. (b) Inter-chain hopping with SOI in the organic Kane-Mele model. The lower panel shows the helical edge state in the energy spectrum of the nanoribbon.
At ambient pressure, α-(BEDT-TTF)2I3 undergoes a metal-insulator transition into an insulating phase due to the charge order (CO) at Tc=135K. This CO phase is suppressed by applying pressure, and it perfectly vanishes at about Pc~1.25GPa, as illustrated schematically in the inset of Fig. 1(a). Figure 1(a) shows the temperature dependence of in-plane resistance measured at several pressures. In the weak CO state around Pc, the resistance shows increase due to CO below Tc~50K, but the insulator-like increase turns to metal-like decrease at lower temperatures as shown by the red dashed circle. In addition, in the Dirac semimetal state above Pc, the resistance exhibits the insulator-like increase at low temperatures below 10 K as indicated by the blue dashed circle. These behaviors have never been explained.
As their mechanisms, we propose the emergence of topological states in this organic system. First, we discuss the possible Chern insulator state in the weak CO state [3]. In the vicinity of CO transition, the NMR measurement by Kanoda's group clarified that not only the charge density but also spin density are modulated breaking TRS, as indicated by the clouds in Fig. 2(a). We assume a pattern of site potential and staggered magnetic flux on the α-(BEDT-TTF)2I3 lattice so as to reproduce the observed potential and magnetic modulations (Fig. 2(a)). We shows that the system becomes a Chern insulator, where gaps open at two Dirac points, under large enough magnetic modulation, and one chiral edge state appears in the gap along each crystal edge (Fig. 2(a)). This is an organic analogue of the Haldane model. The conduction through these edge states explains the anomalous metallic behavior of resistance observed in the weak CO state.
Next, we discuss that the Z2 topological insulator state with small gaps emerges in the Dirac semimetal state under finite SOI [4]. Using first principles calculations, Valenti et al. discussed that SOI in BEDT-TTF compounds could reach 1~2 meV, and finite SOI opens small gaps at Dirac points causing insulating behavior at low temperatures (Fig. 1(b)). We assume a pattern of inter-chain hopping accompanied by SOI on α-(BEDT-TTF)2I3 lattice reflecting the charge disproportionation indicated by the clouds in Fig. 2(b). We demonstrate that gaps open at Dirac points causing resistance increase, and one helical edge state, which is characteristic to the topological insulator, appears in the gap along each crystal edge. This is an organic analogues of the Kane-Mele model. In addition, we generally discuss that a topological insulator appears under finite SOI as long as SOI does not cause any band inversion, based on the Fu-Kane parity product theory (Fig. 1(b)) [5]
References
- [1] F. D. M. Haldane, Phys. Rev. Lett. 61, 2015 (1988).
- [2] C. L. Kane and E. J. Mele, Phys. Rev. Lett. 95, 226801 (2005).
- [3] T. Osada, J. Phys. Soc. Jpn. 86, 123702 (2017).
- [4] T. Osada, arXiv: 1804.09420.
- [5] L. Fu and C. L. Kane, Phys. Rev. B 76, 045302 (2007).