Anisotropic Proton Conductivity Arising from Hydrogen-Bond Patterns in Anhydrous Organic Single Crystals
Mori Group
Proton conduction is a fundamentally important phenomenon not only in living systems but also in solid materials, such as fuel cells. Especially, the development of high proton conducting materials and the elucidation of the conducting mechanism are one of pivotal issues in this research field. Recently, organic acid-base salts have attracted increasing attention as a promising candidate of anhydrous proton conductors, which can be utilized as electrolyte of fuel cell above 100 ºC. In this study, we have successfully disclosed the relationship between proton conductivity and hydrogen-bond (H-bond) interactions in such kinds of organic salts, composed of dicarboxylic acid and imidazole [1].
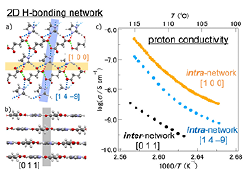
Fig. 1. a) Intra- and b) inter-2D-hydrogen (H)-bonded network [2], and c) anisotropic proton conduction along the [1 0 0], [1 4 –9], and [0 1 1] directions, respectively [1] for an anhydrous proton conductor, imidazolium succinate (Im-Suc). The proton conductivity in intra-network is by two-orders higher than that in inter-network. Moreover, the anisotropic proton conduction in intra-network demonstrates the importance of H-bonded molecular arrangement.
We have grown high-quality single crystals of imidazolium succinate (Im-Suc) or glutarate (Im-Glu) by evaporation method in dehydrated methanol and dehydrated acetonitrile with a typical size of 0.5 × 0.7 × 1.0 mm3 (Im-Suc). As shown in Fig. 1, the crystal is composed of two-dimensional (2D) sheet structures, in which the imidazolium cations (Im) and succinate anions (Suc) are connected with hydrogen bonds (H-bonds) [2]. Two N–H moieties of the Im molecule are connected to carbonyl oxygen atoms of the Suc molecule with dNO of 2.72 Å (red dashed lines) and 2.87 Å (brown dashed lines) and also form short contacts with the adjacent oxygen atoms of the Suc molecule with dNO ~ 3.05, 3.15 Å (blue dashed lines), like bifurcated H-bonds. In addition, a C-H···O short contact (gray dashed lines, dCO ~ 3.08 Å) is observed between the Im and Suc molecules. Furthermore, there is an O-H···O H-bond between the Suc molecules (green dashed lines, dOO ~ 2.49 Å). On the other hand, although the shortest C···C distance (3.39 Å) between the networks is less than the sum of van der Waals radii (3.40 Å), no effective H-bonds are found between these 2D networks (Fig. 1). The shortest N···O distances between the networks are 3.50 and 3.56 Å which are much longer than the sum of van der Waals (vdW) radii of N and O atoms (3.07 Å).
The relationship between the crystal shape and crystallographic axes was determined by X-ray diffraction measurements, where the [1 0 0] and [1 4 –9] directions are within the 2D network and the [0 1 1] is perpendicular to the 2D network (Fig. 1). Therefore, by measuring and comparing the proton conductivity in these three directions, we have revealed the effects of the H-bonding interactions and molecular arrangement on the proton conduction. By the measurement of ac proton conductivity, the complex impedance planes (Cole-Cole plots) gave almost perfect semicircle profiles at each temperature, which means that a single Debye-type relaxation occurs in our single-crystal measurements and thus the obtained conductivity is the intrinsic proton conductivity without contributions from the grain boundaries. Then, we compare the proton conducting properties in these three directions (Fig. 1). First, the proton conductivity σ in the directions parallel to the H-bonding network (σ [1 0 0] and σ [1 4 –9]) and that in the direction perpendicular to the network (σ [0 1 1]) are compared. At around 115 °C, the former two directions (σ [1 0 0] = 4.94 × 10–7 S cm–1 and σ [1 4 –9] = 1.27 × 10–7 S cm–1) provide almost two orders higher conductivity than the latter one (σ [1 0 0] = 3.55 × 10–9 S cm–1). This large difference is maintained in the present temperature range, indicating that the proton conduction occurs more readily in the H-bonding network than in its perpendicular direction.
Next, σ in the two directions parallel to the H-bonding network (σ [1 0 0] and σ [1 4 –9]) are compared. At 115 °C, the [1 0 0] direction has about four times higher σ (4.94 × 10–7 S cm–1) than the [1 4 –9] direction (1.27 × 10–7 S cm–1) and this trend is unchanged in this temperature region. The difference of σ between these two directions (about four times) is much smaller than that between the parallel and perpendicular directions (two orders) shown above. However, this small difference is significant in connection with anisotropy of the proton conduction in the 2D H-bonding network, especially in terms of the intermolecular interactions and molecular arrangement.
Moreover. in order to gain further insight into this relationship between the difference in ΔpKa and the difference in the proton conductivity, we compare the present results with those of the analogues. The 1:1 co-crystals of benzimidazole, an imidazole derivative with pKaH = 5.49, and dicarboxylic acid are a similar type of H-bonded proton conductors, showing σ = ~10–5 S cm–1 in the glutaric acid (pKa1 = 4.34) salt and ~10–4 S cm–1 in the sebacic acid (pKa1 = 4.72) salt in the compressed pellet state at around 77 °C. This result indicates that the latter salt with a smaller ΔpKa of 0.77 (= 5.49 – 4.72) shows a higher proton conductivity than the former salt with a ΔpKa of 1.15 (= 5.49 – 4.34). This trend is similar to that in the present imidazole systems, suggesting that the proton conductivity significantly increases with decreasing ΔpKa. However, the degree of the conductivity increase seems to be somewhat different in the benzimidazole and imidazole systems. Namely, the proton conductivity of the former system is increased one order of magnitude by the ΔpKa difference of 0.38 (= 1.15 – 0.77), whereas that of the latter is increased 2–3 orders of magnitude by the ΔpKa difference of 0.15 (= 2.76 – 2.61) as described before. Thus, simply stated, the proton conductivity of the present imidazole system is more sensitive to the pKa change than that of the benzimidazole system. This difference of the pKa effect on the proton conductivity probably originates from the difference in the molecular structure of imidazole and benzimidazole. Although these two systems have a similar overall crystal structure, the molecular structure difference would modulate the details, such as the intermolecular distances, H-bonding manners, and molecular arrangements, which result in the above-described difference in the pKa effect.
In conclusion, we have revealed the “intrinsic” proton conductivity without grain boundary contributions in acid-base type anhydrous organic single co-crystals, imidazolium succinate (Im-Suc) and imidazolium glutarate (Im-Glu), with a 2D H-bonding network. The obtained conductivities are 1~5 × 10–7 S cm–1 (Im-Suc) and 2 × 10–6 S cm–1 (Im-Glu) within the 2D H-bonding network, which are much higher than those in the perpendicular direction to the 2D network [4 × 10–9 S cm–1 (Im-Suc) and 6 × 10–8 S cm–1 (Im-Glu)]. These results demonstrate that the H-bonds significantly promote proton conduction in this kind of anhydrous materials. Furthermore, we have shown that this proton conduction is 1) more significant in the H-bonds between the acid and base molecules than in those between the acid and its conjugate base and is 2) enhanced by decreasing the difference of pKa between the H-bonded acid and base molecules. In addition, the present results offer the possibility that the molecular motions of imidazole play a crucial role in the proton conduction not only in the 2D H-bond network but also between the networks. The details about the inter-network proton conduction and also the non-Arrhenius-type profile of proton conductivity in the H-bond network are currently under investigation in terms of the molecular dynamics by using solid-state NMR and IR spectroscopies, high-temperature X-ray analysis, and theoretical calculations.
References
- [1] Y. Sunairi, A. Ueda, J. Yoshida, K. Suzuki, and H. Mori, J. Phys. Chem. C, in press.
- [2] J. C. MacDonald, P. C. Dorrestein, and M. M. Pilley, Cryst. Growth Des. 1, 29 (2001).