Pressure induced Bulk Superconductivity in a Layered Transition-Metal Dichalcogenide 1T-Tantalum Selenium
Uwatoko Group
Charge density wave (CDW) and superconductivity (SC) are two low-energy collective excitations in condensed matter physics. The periodic electron density modulations of CDWs and the anisotropic energy gaps at Fermi surface generates multiple commensal electronic orders, either compete or cooperate. The resultant electronic phase diagrams have been devoted extensive efforts by controlling external stimulations to reveal the intrinsic physics.
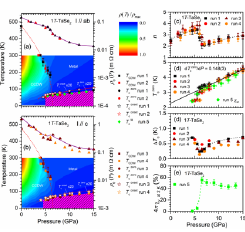
Fig. 1. T-P diagram and the colors describe the evolution of resistivity; the residual resistivity ρ0 is estimated by fitting ρ = ρ0+ATn up to 20 K. The dashed red line, the red line, the triangle points represent the trend of TCDW, Tconset, and ρ0; the parameters versus pressure: (c) the exponent n, (d) Tczero and TcM, (e) ΔTc, (f) 4πχv (2K).
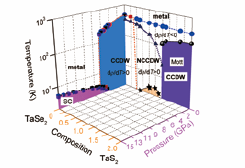
Fig. 2. Phase diagram of 1T-TaSe2 in temperature, pressure, and composition. Here, the dρ/dT is the differential coefficient of resistivity. The red dished line separates the CCDW and NCCDW and the triangle symbols divide the negative and positive dρ/dT.
Layered transition-metal dichalcogenides (TMDs) have been studied for nearly 50 years, but the key factors on the interplay of CDW and SC are far from clear. In one case, the superconducting transition temperature assumes a dome-like shape close to the collapsed CDWs; while in other cases, Tc changes insensitively and/or increases monotonously without domes. In former, the superconducting dome resembles that of unconventional SC neighboring quantum critical point. One scenario is proposed that CDW fluctuation glues superconducting pairs since CDW and SC originate from Fermi surface instabilities and electron-phonon coupling. Howbeit, an opposite scenario is argued that CDW is weakly connected to SC (e.g., 1T-TaS2) in 1T-TMDs, and the dome-shaped superconducting diagram is far from CDW, which tally with the evidences of conventional single-gapped s-wave SC. Additionally, superconducting diagram and the coexisting model of CDW and SC depends on tuning routes even from the same starting point. Some argue that CDW and SC coexist on a macroscopic scale in real space, while others support that the insulating CDW domain walls coexist with superconducting interdomains. It implies that the SCs are distinct in superconducting diagrams generated by different parameters, and the coexisting model of CDW and SC is essential to understand the superconducting mechanism.
1T-TaSe2, with a higher commensurate CDWs transition temperature ~ 473 K and a larger unit-cell volume compared to isostructural compounds, has attracted our attention as a starting point to explore SC and reveal the interplay of CDW instability and SC by pressure. In this work, we report a pressure-driven SC in the vicinity of CCDW in TMDs 1T-TaSe2 by resistivity and ac susceptibility. The superconducting phase enters at 4.5 GPa and bulk SC emerges along with the collapse of CCDW at a critical pressure Pc ~ 6.5 GPa. Higher than Pc, Tc keeps increasing linearly, without a dome-shaped superconducting diagram in our pressure range. Tc reaches ~ 5.3 K at 15 GPa. The comprehensive analysis shows that electronic correlations of CCDW phase open energy gaps, which prohibits coopers pairing; while the superconducting channels and CCDW domain wall coexist in three-dimensions above Pc. The evolutions of Fermi surface and the softening of phonon models under pressure are proposed to explain the monotone increase of Tc. The findings reveal the interplay of CCDW and SC in 1T-TaSe2 by a clean method viz. high-pressure, and shed light on the underlying superconducting mechanism in the relevant systems.
References
- [1] B. S. Wang, Y. Liu, K. Ishigaki, K. Matsubayashi, J. -G. Cheng, W. J. Lu, Y. P. Sun, and Y. Uwatoko, Phys. Rev. B. (rapid) 95, 220501(R) (2017)