Photoinduced Demagnetization and Insulator-to-Metal Transition in Ferromagnetic Insulating BaFeO3 Thin Films
Wadati Group
Control of magnetic states by optical excitations in magnetically ordered materials has attracted considerable attention since the demonstration of ultrafast demagnetization in Ni within 1 ps, explored by time-resolved magneto-optical Kerr effect studies by Beaurepaire et al. [1]. They proposed a phenomenological “three-temperature model” in order to understand the ultrafast demagnetization, which considers three interacting reservoirs of electrons, spins, and lattice, and suggested the importance of direct electron-spin interactions. Here we report on pump-probe time-resolved resonant x-ray reflectivity study of fully oxidized single crystalline BaFeO3 thin films [2], which show unusual behaviors of ferromagnetic and insulating properties with saturation magnetization and a Curie temperature of 3.2 μB/formula unit and 115 K, respectively [3,4]. The investigation of the demagnetization dynamics of insulators allows one to relate electronic structure to magnetic dynamics.
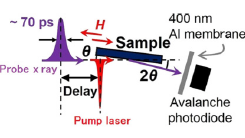
Fig. 1. Geometry of the measurements.
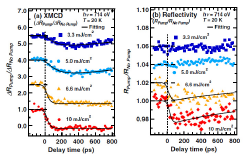
Fig. 2. Time evolution of (a) XMCD intensity and (b) reflectivity of BaFeO3 thin films for various pump laser fluence. All the curves, except for the case of 10 mJ/cm2, are shifted upward for clarity.
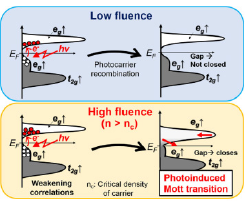
Fig. 3. Mechanism of the insulator-to-metal transition induced by the strong laser excitation.
In order to investigate the magnetic dynamics of ferromagnetic insulating BaFeO3 thin films, we performed timeresolved reflectivity studies at the Femtospex slicing facility at the synchrotron radiation source BESSY II [5], using circularly polarized x-ray pulses. Our experimental method has the advantage that, in one reflectivity experiment, we can probe electronic structure as well as magnetism. The quality of the thin-film samples was confirmed by x-ray diffraction, Fe 2p x-ray absorption spectroscopy, and Fe 2p core-level hard x-ray photoemission spectroscopy measurements by comparing cluster-model calculations, which found that the formal valence of Fe was 4+ [3,4]. The experimental geometry is shown schematically in Fig. 1. We used fixed circular polarization and created magnetic contrast by switching the direction of the magnetic field (H), which was oriented along the sample surface ([010] direction). We recorded specular reflectivity data for two magnetization directions R+ and R−. The average reflectivity R = (R+ + R−)/2 is a measure of the electronic and structural properties, while the magnetic circular dichroism in reflectivity (MCDR) signal DR = (R+ - R−)/2 is a measure of the sample magnetization. A Ti:sapphire laser (wavelength: 800 nm, energy: 1.55 eV) with a pulse width of ∼50 fs was employed as a pump laser with π polarization. The spot size of the pump laser was ∼0.40 mm (horizontal) × 0.25 mm (vertical), and that of the probe x ray was ~0.1 mm × 0.1 mm. The repetition rate of the time-resolved measurement was 3 kHz, limited by the frequencies of the pump laser. The pumped and unpumped signals were obtained alternatively. The time resolution was 70 ps, corresponding to the pulse length of the probe x ray.
Figure 2(a) shows the time evolution of the MCDR intensities for different pump fluences. The vertical axis shows the excited MCDR intensities normalized by the unpumped signal (i.e., ΔRpump /ΔRno pump). Here, the subscript of pump and no pump denote the signals with and without the laser excitations, respectively. The MCDR intensities decrease after the incidence of the pump laser at t = 0. The time evolution of the MCDR intensity shows different behaviors with the change of the pump fluence (F). When F is smaller than 5.0 mJ/cm2, the demagnetization time is relatively slow and magnetization recovery sets in after about 400 ps. When the pump F is larger than 6.6 mJ/cm2, on the other hand, the demagnetization time is quite fast and no recovery of the magnetization can be observed within the first 800 ps. We assign the different behavior of the demagnetization dynamics to a laser-induced insulator-to-metal transition for F ≥ 6.6 mJ/cm2, as discussed in the following. We show the time evolution of the intensity of the average reflectivity in Fig. 2(b), which allows us to investigate the electronic dynamics. The vertical axis shows the excited reflectivity intensities normalized by those without excitation (i.e., Rpump /Rno pump). No pump effects were observed for F ≤ 5.0 mJ/cm2 with our time resolution of 70 ps. Pump effects, on the other hand, were clearly observed for F ≥ 6.6 mJ/cm2.
Figure 3 shows the mechanism of the insulator-to-metal transition induced by the strong laser excitation. When the pump fluence is weaker than 5.0 mJ/cm2, magnetizations in BaFeO3 thin films recover with the time constant of τrecovery ∼ 1000 ps. The time scale of ∼1000 ps can be assigned to heat diffusion needed to cool the sample below the magnetic ordering temperature after electron, lattice, and spin systems have reached thermal equilibrium. Remarkably, the time-resolved reflectivity change for strong pump fluence also shows a recovery on this time scale of τrecovery ∼ 1000 ps, indicating that also here heat diffusion is the relevant mechanism. This latter observation is quite notable, because equilibrium between the electron and lattice temperature should be reached within 1 ps. The slow reopening of the band gap on time scales of ∼1000 ps shows that for high excitation densities we drive the system into a metastable state. As a mechanism for the long lifetime of the metallic state, we consider that hot carriers, generated by the quasiparticle scattering and closing the band gap, prevent it from opening again by reducing electron-electron and electron-phonon interactions.
In summary, we investigated the electronic and magnetic dynamics by time-resolved reflectivity and MCDR measurement on BaFeO3 thin films. When the pump laser fluence is smaller than 5.0 mJ/cm2, a relatively slow demagnetization of τdecay ∼ 150 ps was observed, due to the insulating properties of the ground state in BaFeO3 thin films without any changes in Fe 2p x-ray reflectivity. When the pump laser fluence is stronger than 6.6 mJ/cm2, on the other hand, rapid changes in Fe 2p x-ray reflectivity are observed, which is attributed to a transition into a metallic state, resulting in an unusually fast demagnetization with τdecay < 70 ps. Since BaFeO3 thin films are near the phase boundary of a metal-insulator transition, the insulating phase is quite sensitive to carrier density. Thus, the origin of the insulator-to-metal transition is a photoinduced Mott transition into a metastable state stabilized by screened electron-electron and electron-phonon interactions. Our findings indicate a mechanism for tuning magnetic dynamics in correlated materials, which resembles heat-assisted magnetic switching in metallic magnets. By creating a sufficiently high excitation density, spin-flip scattering channels open up which increase the spin system susceptibility to external manipulation.
References
- [1] E. Beaurepaire, J.-C. Merle, A. Daunois, and J.-Y. Bigot, Phys. Rev. Lett. 76, 4250 (1996).
- [2] T. Tsuyama, S. Chakraverty, S. Macke, N. Pontius, C. Schüßler-Langeheine, H. Y. Hwang, Y. Tokura, and H. Wadati Phys. Rev. Lett. 116, 256402 (2016).
- [3] S. Chakraverty, T. Matsuda, N. Ogawa, H. Wadati, E. Ikenaga, M. Kawasaki, Y. Tokura, and H. Y. Hwang, Appl. Phys. Lett. 103, 142416 (2013).
- [4] T. Tsuyama, T. Matsuda, S. Chakraverty, J. Okamoto, E. Ikenaga, A. Tanaka, T. Mizokawa, H. Y. Hwang, Y. Tokura, and H. Wadati, Phys. Rev. B 91, 115101 (2015).
- [5] K. Holldack, J. Bahrdt, A. Balzer, U. Bovensiepen, M. Brzhezinskaya, A. Erko, A. Eschenlohr, R. Follath, A. Firsov, W. Frentrup, L. Le Guyader, T. Kachel, P. Kuske, R. Mitzner, R. Muller, N. Pontius, T. Quast, I. Radu, J.-S. Schmidt, C. Schüßler-Langeheine, M. Sperling, C. Stamm, C. Trabant, and A. Fohlisch, J. Synchrotron Radiat. 21, 1090 (2014).