Novel Pressure Dependence of the Superconductivity in Non-Centrosymmetric LaNiC2: Evidence of Strong Electronic Correlations
S. Katano and Y. Uwatoko
Since the discovery of the heavy-fermion superconductor CePt3Si [1], superconductivity of non-centrosymmetric systems has attracted great interest. The non-centrosymmetry causes the antisymmetric spin-orbit coupling (ASOC) that leads to the indistinguishability of spin-singlet and spin-triplet electron pairings. LaNiC2 forms a non-centrosymmetric structure (space group: orthorhombic Amm2), and exhibits superconductivity below the transition temperature Tc of about 3 K. On this superconductivity a recent muon spin relaxation (μSR) experiment indicated the breaking of the time-reversal symmetry (TRS) in the superconducting state, which leads to non-unitary p-wave spin-triplet pairings [2]. However, a NQR study suggested an enhancement of the nuclear spin relaxation rate (1/T1) just below Tc, implying a conventional BCS-type superconductor [3]. Therefore its superconducting mechanism is still unclear. Furthermore, this system is not magnetic, and thus it has been considered that its electronic correlations would be weak in contrast with other heavy fermion systems. To get further information on this superconductivity, pressure effects on the superconducting state of LaNiC2 have been studied.
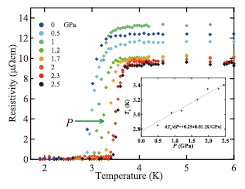
Fig. 1. Electrical resistivity under high pressures up to about 3 GPa. The inset shows that pressure enhances the superconducting transition temperature Tc substantially.
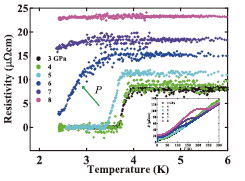
Fig. 2. Electrical resistivity under high pressures up to 8 GPa. The inset indicates that the resistivity at high temperatures shows an anomaly with a clear hump, implying an emergence of a new state.
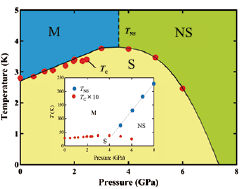
Fig. 3. P-T phase diagram of LaNiC2. The mark M and S denote metal and superconductivity, respectively; and NS indicates ‘a new state’ induced under pressure. In the inset, the superconducting transition temperatures are multiplied by the factor of 10.
Figure 1 shows the temperature dependence of the electrical resistivity of LaNiC2 under high pressures up to 3 GPa. Under these pressures, Tc is enhanced greatly at the rate of 0.25 K/GPa. For normal conventional metals pressure contracts their lattices, and generally the electron density D(EF) at the Fermi energy EF decreases. In the BCS theory, therefore, Tc would decrease with increasing pressure. The results obtained here thus suggest that the superconductivity of this system is possibly changed under high pressure by some novel mechanism.
Above the pressure of 3 GPa, the superconductivity enhanced once is heavily suppressed. As shown in Fig. 2, the pressure of 8 GPa extinguishes its superconductivity completely. At the higher temperatures, on the other hand, the resistivities above 5 GPa show clear anomalies over 100 K, as displayed in the inset of Fig. 2. The temperature observed as an anomalous hump increases with pressure up to room temperature at the rate of 51.5 K/GPa. These results show that a new state (NS) is evidently induced under high pressures. With such an emergence of the NS state, the superconductivity is intensely suppressed. Figure 3 shows the phase diagram obtained. It is noted that this diagram shows a dome for Tc but is mirror symmetric to those of many other systems like heavy-fermion and high-Tc superconductors.
For this NS state it remains that some RNiC2 (R = rare-earth) systems show charge density wave (CDW) states at high temperatures. Thus, it might be natural that the anomalous hump observed at the temperatures over 100 K originates from the phase transition to the CDW state. This newly induced state is enhanced strongly under high pressures; further, that is quite robust up to the highest pressure of 30 GPa investigated in this work.
With the disappearance of the superconductivity a new state (NS) with a high energy scale is dramatically emerged. These results indicate that the system is not a simple normal metal but is highly correlated with strong electronic interactions such as CDW, which correlations may contribute to the superconductivity of this unique system [4].
References
- [1] E. Bauer et al., Phys. Rev. Lett. 92, 027003 (2004).
- [2] A.D. Hillier, J. Quintanilla, and R. Cywinski, Phys. Rev. Lett. 102, 117007 (2009).
- [3] Iwamoto et al., Phys. Lett. A 250, 439 (1998).
- [4] S.Katano et al., Phys. Rev. B 90, 220508(R) (2014).