Insulator-Metal Transition in Perovskite Cobalt Oxides at Ultrahigh Magnetic Fields
Y. Matsuda and Takeyama Groups
Phase transitions between metal and insulator are a subject that has been fascinating scientists for a long time. According to the one-electron approximation, materials whose energy band is partially occupied by electrons become metal. In 1930’s, however, it had already been pointed out that some materials don’t obey this rule. Nowadays, it is known that the electron-electron interaction plays an important role for making materials insulating and exotic phenomena such as non-Fermi liquid behavior and unconventional superconductivity emerge in the vicinity of the metal-insulator transition. The insulating phases of many materials appear along with interesting magnetic features such as antiferromagnetic order, spin gap formation and spin state transition [1]. Possible control of metal-insulator transitions by magnetic field is one of the most intriguing research subjects.
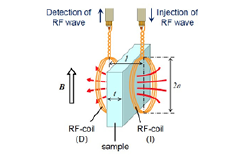
Fig. 1. Schematic view of a pair of coils and a sample for the high-frequency contactless electrical resistivity measurement. The coils are for injection and detection of the electromagnetic wave, respectively. The frequency range employed is 240 – 400 MHz. The magnetic field dependence of the electrical resistivity is obtained as the field variation of the transmission of the electromagnetic wave through the sample.
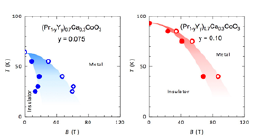
Fig. 2. The magnetic field-temperature (B-T) phase diagram determined by the magnetoresistance experiment. The higher field phase boundary (open circles) is obtained in the field-increasing process of the pulsed magnetic field and the lower field phase boundary (filled circles) by the field-descending process. The hysteretic behavior is the evidence of the first-order phase transition. Judging from the width of the hysteresis, the first order nature is more prominent in y=0.075 (a) than y=0.10 (b).
In the present work, we studied the magnetic-field-induced insulator–metal transition in perovskite cobalt oxides (Pr1-yYy)0.7Ca0.3CoO3 (y = 0.075, 0.10). (Pr1-yYy)1-xCaxCoO3 is obtained by substitution of Y for a part of Pr in Pr1-xCaxCoO3 that shows a sharp first-order spin state (SS) transition of Co only when x is close to 0.5. The striking point is that the metal-insulator (MI) transition and the SS transition take place at the same time at 90 K. It is recently found that (Pr1-yREy)1-xCaxCoO3 (RE= rare-earth element) also exhibits the first-order MI-SS transition even when x is different from 0.5 and the transition temperature can be tuned by x and y [2]. We employed polycrystals of (Pr1-yYy)0.7Ca0.3CoO3 (y=0.075, 0.10) that were grown by a solid-state reaction method. The MI transition temperatures (TMI) at zero magnetic field are 64 and 93 K for y=0.075 and 0.10, respectively. One can expect that a magnetic field induces the metallic phase at low temperatures when the Zeeman energy overcomes the energy gap between the different spin states. In order to realize the phase transition, ultrahigh magnetic fields in the range of 100 T will be required because the TMI is rather high.
The single-turn coil method is utilized for generation of the pulsed magnetic fields over 100 T. We used the high frequency contactless technique to measure the electrical resistivity of (Pr1-yYy)0.7Ca0.3CoO3 (y=0.075, 0.10). The sample is sandwiched by two small coils; one coil is for generation of electromagnetic wave and another is for detection of the wave after transmission of the sample. The measured intensity of the transmitted wave is a function of the electrical resistivity of the sample (e.g., the transmission is small when the resistivity is low owing to the shielding effect by the eddy current) and we can obtain the magnetoresistance from the magnetic field variation of the transmission. The schematic view of the coils and sample is shown in Fig. 1. This method was originally developed by Sakakibara et al. [3]. We modified the dimensions and used higher frequencies (240 – 400 MHz) to optimize the method for the present work.
As results of the experiment, we found that the transmission showed an abrupt decrease at certain magnetic field when the temperature is lower than TMI, suggesting the insulator–metal transition occurs by magnetic fields. The phenomenon was observed in both samples but the transition magnetic field depends on sample and temperature. Figure 2 shows the magnetic field-temperature (B-T) phase diagram for the MI-SS transition in (Pr1-yYy)0.7Ca0.3CoO3 (y=0.075, 0.10) [4] . The marks denote the transition magnetic fields at different temperatures. The MI-SS transition is the first-order transition and we observed significant hysteretic behavior. The higher-field values in Fig. 2 are obtained in the field-increasing process and the lower-field values in the field-descending process. Considering the Clausius–Clapeyron relation, the obtained phase boundary indicates that the entropy change is important for the transition. However, the entropy change expected from only the SS transition cannot explain the phase boundary. Moreover, recent discovery of the valence change of Pr at the MI-SS transition [5] suggests that not only Co but also Pr plays an important role for the MI transition. Although the microscopic understanding of the MI-SS transition is still in controversy, the obtained B-T phase diagram sheds new light on this problem.
References
- [1] M. Imada, A. Fujimori, and Y. Tokura, Rev. Mod. Phys. 70, 1039 (1998).
- [2] T. Naito, H. Sasaki, and H. Fujishiro, J. Phys. Soc. Jpn. 79, 034710 (2010).
- [3] T. Sakakibara, T. Goto, and N. Miura, Rev. Sci. Instrum. 60, 444 (1989).
- [4] S. Lee, Y. H. Matsuda, T. Naito, D. Nakamura, and S. Takeyama, J. Phys. Soc. Jpn. 84, 044703 (2015).
- [5] H. Fujishiro, T. Naito, S. Ogawa, N. Yoshida, K. Nitta, J. Hejtmánek, K. Knížek, and Z. Jirák, J. Phys. Soc. Jpn. 81, 064709 (2012).