One-Hundred-Million-Atom Electronic Structure Calculations on the K Computer
T. Hoshi
In the current (peta-scale) or next generation (exa-scale) computational physics, a crucial issue is ‘Application-Algorithm-Architecture co-design’ or inter-disciplinary collaborations among physics, applied mathematics and the high-performance computation field.
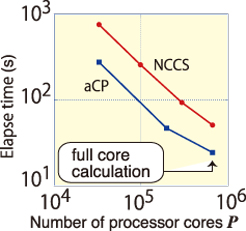
Fig. 1. Benchmark for the parallel efficiency (‘strong scaling’) on the K computer with one hundred million atoms. [1,2] The calculated systems are amorphous-like conjugated polymer (aCP), poly-(9,9 dioctyl-fluorene) and sp2-sp3 nano-composite carbon solid (NCCS).
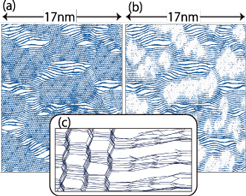
Fig. 2. Nano-domain analysis of NCCS visualized with the πCOHP [4]. The sp2 (graphite-like) and sp3 (diamond-like) domains are visualized in (a), while only the sp2 domains are visualized in (b). A closeup of an sp2- sp3 domain boundary is shown in (c).
Here we report that one-hundred-million atom (100-nm-scale) electronic structure calculations were realized on the K computer [1,2]. The methodologies were based on the co-design. In particular, the novel iterative (Krylov-subspace) algorithms were constructed for the generalized shifted linear equations ((zS-H)x=b), instead of the conventional generalized eigen-value equation (Hy=eSy) (See [3,4] and reference therein). The methods give the real-space Green’s function, instead of eigen states. These solvers were implemented in our order-N calculation code ELSES (http://www.elses.jp/) with modeled (tight-binding) systems based on ab initio calculations. These solver algorithms are purely mathematical and applicable to large-matrix problems in many computational physics fields beyond electronic structure calculations.
In Fig. 1, the calculations show high parallel efficiency (‘strong scaling’) with up to the full core calculations of the K computer for one-hundred-million-atom (100-nm-scale) systems [1,2]. The calculated systems are amorphous-like conjugated polymer (aCP), poly-(9,9 dioctyl-fluorene) with N=102,238,848 atoms and sp2-sp3 nano-composite carbon solid (NCCS) with N=103,219,200 atoms. The high parallel efficiency stems not only from the fundamental mathematical theory but also from detailed techniques, such as programing techniques for saving memory and communication costs and a parallel file I/O [4].
An application study with NCCS is picked out [4]. The study is an early-stage one on the formation process of the nano-polycrystalline diamond (NPD), a novel ultrahard material [5]. NPD is obtained by direct conversion sintering process from graphite under high pressure and high temperature and has characteristic 10-nm-scale lamellar-like structures. NPD is of industrial importance for its extreme hardness and strength and Sumitomo Electric Industries. Ltd. began commercial production from 2012. Our simulation is motivated by the investigation of possible precursor structures in the formation process of NPD and the structures should be nano-scale composites of sp2 (graphite-like) and sp3 (diamond-like) domains. Figure 2 shows the nano-domain analysis on the NCCS. The structure is a result of our simulation. The π-type crystalline orbital Hamiltonian population (πCOHP) analysis [4], an analysis method based on the Green’s function, was used so as to distinguish the sp2 and sp3 domains. The analysis clarifies shapes of domains and structure of domain boundaries from huge electronic structure data and gives theoretical foundations of the composite.
General future aspects are (i) large-scale calculation methods for optical and transport properties and (ii) applications to various systems, such as organic materials.
The fundamental mathematical theory was constructed in the collaboration with applied mathematics researchers; T. Sogabe (Aichi Prefectural University) and S.-L. Zhang (Nagoya University) [3]. The code for massive parallelism was developed with the supercomputers, the systems B and C, at ISSP and the K computer was used in the research proposals of hp120170, hp120280 and hp130052.
References
- [1] T. Hoshi et al., JPS Conf. Proc. 1, 016004 (2014).
- [2] T. Hoshi et al., Proceedings of Science, in press (Preprint : http://arxiv.org/abs/1402.7285/)
- [3] T. Hoshi et al., J. Phys.: Condens. Matter 21, 165502 (2012).
- [4] T. Hoshi et al., J. Phys. Soc. Jpn. 82, 023710 (2013).
- [5] T. Irifune et al., Nature 421, 599 (2003).