Nano-magnetism in Relaxor Magnet LuFeCoO4
Masuda Group
Dielectric property exhibiting an enhanced permittivity in wide temperature range is known as relaxor ferroelectricity and it has attracted great interest in the field of basic and applied physics. In the relaxor system Polar Nanoregions (PNRs), where coherent polarizations in nano-scale domains are randomly oriented in the bulk crystal, play important role. So far disordered perovskite oxides such as Pb(Mg1/3Nb2/3)O3, Pb(Sc1/2Ta1/2)O3, etc., have been extensively studied [1] and all of them are purely dielectric and non-magnetic. Here natural question arises: what would happen if the relaxor system contains magnetic ions? One of our group members answered to the question in his pioneering work on a relaxor magnet, 2/3BiFeO3-1/3BaTiO3 [2]; appearance of a new type of nano-magnetism where superparamagnetic moments are induced limitedly inside the PNRs. For further understanding of relaxor magnets we study a new compound LuFeCoO4 by combination of bulk properties measurements and neutron diffraction technique. Our study reveals the relationship between PNRs and magnetic correlation, and establishes the magnetic and dielectric phase diagrams of the relaxor magnet as shown in Fig. 1(a) [3].
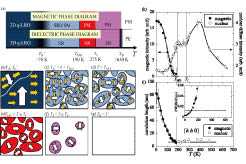
Fig. 1. (a) Magnetic phase diagram (upper panel) and dielectric phase diagram (lower panel). PM, SM, NR, ER, and PE mean paramagnetism, superparamagnetism, nonergodic relaxor, ergodic relaxor, and paraelectric, respectively. (b)-(g) The relationship between PNR and magnetic correlation at various temperatures. White arrows are electric polarization and yellow arrow are weak-ferromagnetic moments. (h) Temperature dependence of magnetic and nuclear diffuse intensities. (i) The temperature dependencies of magnetic and nuclear correlations.
LuFeCoO4 is a two-dimensional (2D) triangular spin system where Fe3+ and Co2+ ions are randomly positioned on the vertices of the triangles. Temperature dependence of the permittivity constant showed a typical behavior of a conventional relaxor. The result suggested the existence of PNRs and it is supported by neutron diffuse scattering. Magnetic susceptibility showed a weak-ferromagnetic component and magnetization showed superparamagnetic behavior at T < 190 K. With further decreasing the temperature the susceptibility showed a well-defined anomaly at 70 K and the magnetization showed hysteretic behavior at the base temperature, indicating a realization of magnetic order with a weak-ferromagnetic component.
The nuclear scattering results at T > 190 K in Fig. 1(h) show typical behavior of relaxor systems. At 190 K < T < 275 K, the PNRs are freezed and static. With decreasing the temperature at T = 190 K the nuclear diffuse intensity begins to decrease and simultaneously the magnetic peak appears. These results mean that the size of the PNRs increases and, synchronizedly, magnetic correlation develops. The magnetic correlation length at 190 K is 7 nm and it is the same as that of nuclear scattering as shown in Fig. 1(i).
Based on the bulk property measurements and neutron diffraction experiments, we propose a superparamagnetic model that a weak ferromagnetic correlation is, in the temperature range of 190 K < T, developed in the crystallographic c plane inside the PNRs as schematized in Fig. 1(d). Outside the PNRs the nuclear correlation is incoherent and so is the magnetic correlation. Spin moments in the PNRs behave as superparamagnetic moments and those outside the PNRs do as conventional paramagnetic spins. At the temperature TMD ~ 190 K the magnetic correlation achieves long-ranged inside the PNRs. With decreasing the temperature the domain size of PNR increases and consequently the accompanied magnetic domain increases as described in Fig. 1(c). At the TN' = 70 K a magnetic correlation in 2D and dielectric correlation in 3D are both in quasi-long-range order as schematized in Fig. 1(b). Meanwhile at T ≥ TMD the magnetic order is absent as shown in Figs. 1(e)-(g). The proposed model consistently explains both bulk properties and neutron scattering results.
References
- [1] A.A. Bokov and Z.-G. Ye, J. Mater. Sci. 41, 31 (2006).
- [2] M. Soda et al., J. Phys. Soc. Jpn. 80, 043705 (2011).
- [3] M. Soda et al., submitted to Phys Rev. B.