Stability of Quantum Hall Ferromagnetic Phase under High Magnetic Fields in the Organic Dirac Fermion System
Osada Group
A layered organic conductor α-(BEDT-TTF)2I3 under high pressure (>1.5 GPa) is a multilayer Dirac fermion system, in which 2D massless Dirac fermion layers stack with weak interlayer coupling, and the Fermi level is fixed at the Dirac point. Such an undoped 2D Dirac fermion system shows the ν = 0 quantum Hall (QH) effect at sufficiently high magnetic fields resulting from the spontaneous symmetry breaking (SSB) of four-fold (spin and valley) degeneracy of the singular n = 0 Landau level. Generally, two kinds of the ν = 0 QH state are possible in the Dirac systems (Fig. 1): One is the QH ferromagnet (QHF) state with the metallic helical edge state consisting of a pair of n = 0 QH chiral edge states with opposite spin and chirality. The other is the QH insulator (QHI) state with no edge state and some real-space structure. In this way, the high-field ground state is one of the key issues of the physics of the Dirac fermion system.
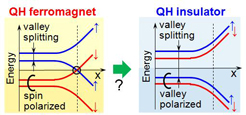
Fig. 1. Schematic energy dispersion of QH edge states in the QHF (left) and QHI (right) states. If the valley splitting becomes larger than the spin splitting in high magnetic fields, the QHF-QHI transition might occur. The helical edge channel (indicated by a circle) in the QHF phase disappears at the QHI phase.
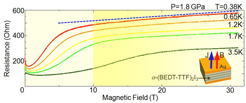
Fig. 2. High-field interlayer magnetoresistance of 2D organic Dirac fermion system α-(BEDT-TTF)2I3. No sign of the QHF-QHI transition was observed.
In graphene, the typical 2D Dirac fermion system, the high-field ground state (the ν = 0 QH state) is believed to be the QHI phase. In contrast, in α-(BEDT-TTF)2I3, the high-field ground state is considered to be the QHF phase. Its interlayer magnetoresistance shows anomalous saturation at high fields. We illustrated that this saturation resistance is scaled not by the sectional area of sample crystals but by sample perimeter. This fact directly means that the metallic surface transport is dominant in the saturating region, and it strongly suggests that the QHF phase with the metallic helical edge state is realized. However, recently, Kanoda et al. observed the fine structures of the NMR lineshape at higher fields (B > 15 T), which suggests the appearance of some real-space structure accompanied by the QHI. Their result shows the possibility of transition from the QHF to the QHI in higher field region.
To confirm this possibility experimentally, we performed high-field transport measurement up to 31 T using the National High Magnetic Field Laboratory at Tallahassee, USA. Since no surface transport exists in the QHI phase, rapid increase of the interlayer resistance can be expected at the QHF-QHI transition. As shown in Fig. 2, the interlayer resistance shows increase reflecting the activated bulk transport at low fields, and it switches to weak increase at higher fields up to 31 T. This saturation is caused by the surface transport via helical edge state. We can see no anomaly in the saturating region, especially around 15 T. This result means that the QHF state survives up to 31 T with no QHF-QHI transition suggested by the NMR study.
The pure QHF phase is spatially uniform state. To explain the NMR result, it might be necessary to consider the mixture of spin and valley degree of freedom.