Evolution of c-f Hybridization and Two Component Hall Effect in β-YbAlB4
Nakatsuji Group
Several recent studies of the mixed-valence compound β-YbAlB4 have revealed the remarkable properties which are seemingly contradictory to one another within a conventional understanding of f-electron intermetallics. β-YbAlB4 is the first Yb-based material in which highly renormalized electronic quasiparticles (with effective masses > 100 times larger than the bare electronic mass) superconduct [1, 2]. In addition, β-YbAlB4 was found to have a quantum critical point at exactly zero magnetic field and zero pressure [3], suggesting the vanishing of an energy scale associated with an ordered electronic state, such as magnetism. On the other hand, in apparent contradiction to these low temperature properties, the Yb f-moment in β-YbAlB4 shows strong valence fluctuations [4] (the Yb valence is +2.75) and has a high Kondo temperature (TK ~ 200 - 300 K) [1, 3]; behaviors that usually lead to a Fermi liquid ground state with weakly renormalized quasiparticles. A study of the Hall effect sheds new light on the evolution of the electronic structure of β-YbAlB4 and suggests how these behaviors can coexist [5].
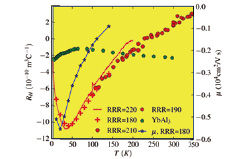
Fig. 1. The Hall coefficient (RH) of β-YbAlB4 vs temperature (T) for several different quality samples compared to a mixed valent compound YbAl3 (left-hand axis). β-YbAlB4 shows a strong temperature dependence characteristic for materials with incoherent skew-scattering from localized moments as expected when the Kondo interaction dominates. The Hall mobility μ obtained from the two band analysis is also shown (right-hand axis).
The Hall effect measurements using high quality crystals of β-YbAlB4 found that the Hall coefficient has strong temperature dependence and a minimum at T = 40 K (see Fig. 1), that bears a close similarity to Kondo rather than mixed-valence systems. The usual interpretation of this result would put the Kondo temperature at 40 K rather than 200 - 300 K, as was measured by the longitudinal resistivity. To answer the question of how β-YbAlB4 can appear to have two Kondo temperatures separated by almost an order of magnitude, we suggested a two component Hall effect, which was supported by the magnetic field dependence of the Hall resistivity.
As the temperature is lowered below 100 K the Hall resistivity becomes non-linear; a careful analysis showed that the field dependence can be explained by the material having two field independent Hall coefficients, the combination of which leads to a non-linear Hall resistivity. Furthermore, this analysis allowed us to show that the mobility of the material strongly increases approaching the minimum at 40 K; thus demonstrating that the minimum in the Hall coefficient is indeed due to the onset of coherent transport in a second component of the electronic transport that has just 10% of the total carrier density.
The reason that these two components have such different Kondo temperatures is suggested by the Fermi surface of β-YbAlB4 together with a recent theoretical analysis showing that the hybridization between conduction electrons and f-moments may vanish at certain points in momentum space [6]. These nodal points lead to a large difference in hybridization strength and thereby a different Kondo temperature between the two Fermi surfaces, one of which passes close to the nodal region and the other of which is well separated.
These results therefore suggest that the emergent second component arises from the Fermi surface that lies close to the region of vanishing hybridization that may be responsible for the quantum critical and superconducting behavior observed at low temperature. This scenario is consistent with a recent theoretical work which provides a phenomenological model for quantum criticality in β-YbAlB4 [6].
References
- [1] S. Nakatsuji, K. Kuga, Y. Machida, T. Tayama, T. Sakakibara, Y. Karaki, H. Ishimoto, S. Yonezawa, Y. Maeno, E. Pearson, G. G. Lonzarich, L. Balicas, H. Lee, and Z. Fisk, Nature Physics 4, 603 (2008).
- [2] K. Kuga, Y. Karaki, Y. Matsumoto, Y. Machida, and S. Nakatsuji, Phys. Rev. Lett. 101, 137004 (2008).
- [3] Y. Matsumoto, S. Nakatsuji, K. Kuga, Y. Karaki, N. Horie, Y. Shimura, T. Sakakibara, A. H. Nevidomskyy, and P. Coleman, Science 331, 316 (2011).
- [4] M. Okawa, M. Matsunami, K. Ishizaka, R. Eguchi, M. Taguchi, A. Chainani, Y. Takata, M. Yabashi, K. Tamasaku, Y. Nishino, T. Ishikawa, K. Kuga, N. Horie, S. Nakatsuji, and S. Shin, Phys. Rev. Lett. 104, 247201 (2010).
- [5] E. C. T. O’Farrell, Y. Matsumoto, and S. Nakatsuji, Phys. Rev. Lett. 109, 176405 (2012).
- [6] A. Ramires, P. Coleman, A. H. Nevidomskyy, and A. M. Tsvelik, Phys. Rev. Lett. 109, 176404 (2012).