Quantum Monopolar Fluctuations in the Exchange-based Spin Ice System Pr2Zr2O7
Nakatsuji Group
Spin ice is a magnetic analogue of H2O ice that harbors dense static disorder. Dipolar interactions between classical Ising spins on pyrochlore lattice yield a frozen frustrated state with residual configurational Pauling entropy [1]. Mimicking the formation of an H3O+−OH− electric dipole in water ice, a spin flip from the spin ice manifold fractionalizes into a pair of emergent magnetic monopolar quasi-particles with Coulomb attraction. In classical spin ice, monopole dynamics is diffusive −only activated thermally or by external magnetic field. The classical nature of Ising spins precludes attainment of thermal equilibrium at temperatures below the effective nearest neighbor energy scale Jff. Introducing quantum fluctuations is of great interest as it enhances dynamics and might allow coherent propagation of magnetic charge (Fig. 1), much as spinons in one dimensional quantum magnets.
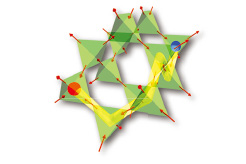
Fig. 1. Schematic illustration of magnetic monopolar quasi-particle with quantum dynamics. Red arrows denote magnetic moment. Red and Blue spheres indicate a pair of magnetic monopoles.
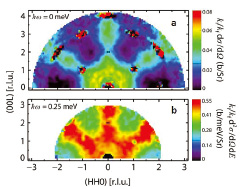
Fig. 2. Spin ice correlations and quantum dynamics in Pr2Zr2O7 probed through elastic and inelastic neutron scattering. (a) Elastic Q-map at 0.1 K with pinch points at (002), (111), and (-1-11). 22 K data was subtracted as a high-temperature background to cancel elastic nuclear scattering processes at Bragg peak. (b) Inelastic Q-map at ħω = 0.25 meV and T = 0.1 K after subtracting the corresponding data at 15 K as background [3].
Here, we report the experimental observation of spin ice correlations and quantum dynamics in a new class of spin ice based on exchange interactions, Pr2Zr2O7 [3]. We have succeeded in growing high quality single crystals at ISSP, which are stoichiometric to the 1-2% level. Inelastic neutron scattering measurements revealed that the crystalline electric field (CEF) ground state of Pr3+ is magnetic doublet with <111> Ising anisotropy, as in classical spin ice systems. AC-magnetic susceptibility and specific heat data show activated dynamics and residual entropy below 0.1 K, again similar to the behavior in classical spin ice systems. However, Curie-Weiss behavior in the temperature dependence of magnetic susceptibility for T < 10 K indicated an effective moment µeff = 2.5(1)µB that is four times smaller than for dipolar spin ice Dy2Ti2O7 where µeff = 10µB. Correspondingly, Pr2Zr2O7 displays an antiferromagnetic (AFM) Weiss temperature θCW = −1.4(1) K compared to the ferromagnetic (FM) θCW ≈ +0.5 K for dipolar Dy2Ti2O7.
Quasi-static spin correlations were investigated using elastic neutron scattering and the result at T = 0.1 K is shown in Fig. 2(a). Sharp pinch point features near (111) and particularly (002), bear evidence of a divergence free two-in two-out spin configuration on each tetrahedron. Indeed, the elastic Q-map resembles a classical Monte Carlo simulation for an exchange only model, which indicates dominant FM superexchange interactions in Pr2Zr2O7. θCW is however, negative and this suggests the exchange Hamiltonian includes AFM transverse terms that induce quantum dynamics. Figure 2(b) shows the Q-map of inelastic scattering at ħω = 0.25 meV and T = 0.1 K. While the overall pattern resembles the nominally elastic scattering (Fig. 2a), the pinch points have vanished. Excited states thus differ from the ground state by the appearance of tetrahedra that violate the ice rule or in other words by the presence of magnetic monopoles, furnishing evidence for magnetic monopolar quantum fluctuations. Such inelastic scattering accounts for >90% of the magnetic scattering cross section at 0.1 K, showing that magnetism in Pr2Zr2O7 is dominated by quantum fluctuations. Our observation in Pr2Zr2O7 is unlike any previously documented in an insulating crystalline magnet. The interplay between monopolar quantum dynamics and itinerant electrons may play an important role in the isostructural Kondo lattice system Pr2Ir2O7 [4].
References
- [1] S. T. Bramwell and M. J. P. Gingras, Science 294, 1495 (2001).
- [2] C. Castelnovo, R. Moessner, and S. L. Sondhi, Nature 451, 42 (2008).
- [3] K. Kimura, S. Nakatsuji, J.-J. Wen, C. Broholm, M. B. Stone, E. Nishibori, and H. Sawa, to be published in Nature Communications (2013).
- [4] Y. Machida, S. Nakatsuji, S. Onoda, T. Tayama, and T. Sakakibara, Nature 463, 210 (2010).