Surface Magnetotransport in Quantum Hall Ferromagnetic Phase in the Organic Dirac Fermion System
Osada Group
In the 2D massless Dirac fermion systems with charge neutrality, the ν=0 quantum Hall (QH) state appears at the high-field quantum limit, resulting from the breaking of four-fold (spin and valley) degeneracy of the n=0 Landau level. Two kinds of ν=0 QH states appear depending on the ratio of spin splitting and valley splitting: One is the spin-unpolarized QH insulating phase, and the other is the spin-polarized QH ferromagnetic phase accompanied by the metallic edge state consisting of a pair of n=0 QH edge states with opposite spin and chirality (helical edge state). The high-field ground state is one of the key issues of the physics of the Dirac fermion system. In undoped graphene, it has been believed that the high-field ground state is the QH insulator.
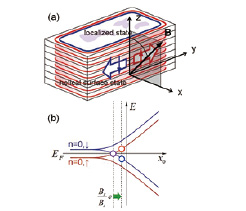
Fig. 1. (a) Helical edge state surrounding the ν=0 QH ferromagnet. (b) Edge state dispersion around the layer edge. Interlayer tunneling causes the shift of x0.
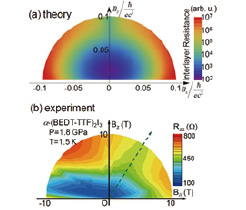
Fig. 2. Interlayer resistance as a function of strength and orientation of magnetic fields. (a) calculation. (b) experiment in α-(BEDT-TTF)2I3.
On the other hand, the Q2D Dirac fermion system, in which 2D massless Dirac layers stack with weak interlayer coupling, is realized in a layered organic conductor α-(BEDT-TTF)2I3 under pressures P>1.5GPa. We found the experimental evidences that the high-field ground state is the QH ferromagneric phase (Fig.1(a)) in α-(BEDT-TTF)2I3 in contrast to graphene. In α-(BEDT-TTF)2I3, the interlayer magnetoresistance shows anomalous saturation at high fields. The saturation resistance is scaled not by the sectional area of sample crystals but by sample perimeter. These experimental facts strongly suggest the interlayer surface transport due to the helical edge state on side surfaces of crystals. The existence of the helical edge state directly means the QH ferromagnet.
We have considered the mechanism of interlayer surface transport due to helical edge state. Since the helical edge state is not topologically protected, transport along the helical edge channel must be diffusive due to spin-inversion scattering. So, we can assume that the interlayer tunneling occurs less frequently than the scattering on the single layer edge. In this case, the interlayer surface transport is dominated by the single tunneling process between the edge states on neighboring two layers. The selection rule of this tunneling leads the shift of center coordinate x0 under finite in-plane magnetic field Bx as shown in Fig.1(b). Therefore, the interlayer tunneling is allowed only when the magnetic field is parallel to the side surface of the crystal (Bx =0).
Figure 2(a) shows the calculated interlayer resistance Rzz, which includes the bulk contribution, as a function of strength and orientation of magnetic fields. We can see that Rzz shows the saturation when the magnetic field is swept in the vertical direction, but shows monotonous increase in other directions. Fig. 2(b) shows the measured interlayer resistance Rzz in α-(BEDT-TTF)2I3. The saturation occurs when the magnetic field was parallel to the stacking direction. Observed features are well explained by the calculation. This agreement also indicates the appearance of the QH ferromagnetic phase with the helical edge state in α-(BEDT-TTF)2I3.