A Novel Method to Verify Spin Diffusion Length
Otani and Kato Groups
Spin relaxation and spin dephasing are the central issues in fields of quantum information and spintronics as they determine how far an electron can transfer spin information, i.e., the spin diffusion length. The spin diffusion length is thus an essential parameter in terms of application in future spintronics devices. However, there is no well-established method for obtaining this length; values reported so far differ greatly depending on the experimental method employed, the number of variables involved in the definition of the spin diffusion length and so on. Since the spin diffusion length determines the spin Hall angle, which is one of the most important physical quantities in spintronics, it is of great importance to evaluate this length correctly.
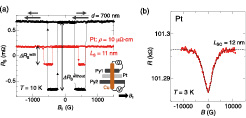
Fig. 1. (a) Nonlocal spin valve (NLSV) signal with a 20 nm thick Pt wire (∆RSwith) in between two Permalloy (Py) wires. As a reference signal, we also plot the NLSV signal without the Pt wire (∆RSwithout). The magnetic field is applied parallel to the Py wires. From the ratio of ∆RSwith/∆RSwithout, the spin diffusion length of Pt can be evaluated (LS = 11±2 nm). A pair of arrows on the top indicates the magnetizations of Py1 and Py2. The inset shows the schematic of our lateral spin valve device. (b) WAL curve of a 20 nm thick Pt wire measured at T = 3 K. In this case, the magnetic field is applied perpendicular to the plane. The broken line is the best fit of Hikami-Larkin-Nagaoka formula. From the fitting, the SO length of Pt can be obtained (LSO = 12±3 nm).
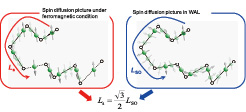
Fig. 2. Spin diffusion lengths measured with ferromagnets (LS; left) and obtained from WAL measurement (LSO; right). The relation of the two length scales (LS = √3/2 LSO) has been experimentally verified
In a conventional method, a lateral spin valve structure where a weak spin-orbit (SO) material such as Cu is bridged by two ferromagnets is used to determine the spin diffusion length. On the other hand, this conventional method cannot be applied to a strong SO material such as Pt, since the spin diffusion length is in general of the order of nanometers. One of the ways to obtain such a short spin diffusion length is to use the spin absorption technique as shown in Fig. 1(a) [1]. However, there was a big debate about how to evaluate the spin diffusion length of a strong SO material [2] because there are several parameters that determine the spin diffusion length, which hinders straightforward evaluation.
Otani group in collaboration with Kato group have discovered a new way to evaluate the spin diffusion length [1]. In the new method, the spin diffusion length can be evaluated just by measuring very precisely the resistance of a SO material (see Fig. 1(b)). In metals, electrons are weakly localized at temperatures approaching absolute zero. When there is a finite SO interaction, the weak localization changes into weak antilocalization (WAL) and the magnetoresistance strongly depends on the SO interaction. In the present work, we focused on this dependency and obtained the spin diffusion lengths of several materials such as Cu, Ag and Pt. The values obtained with the new method are quantitatively consistent with those from the conventional methods. Since the new method reduces the number of variables involved in determining spin diffusion length, a more accurate value can be calculated. In addition, it has been shown theoretically that the spin diffusion length in a localized state and under ferromagnetic conditions varies slightly (by a factor of √3/2). We have experimentally verified for the first time the relationship between the spin diffusion length in a localized state and under ferromagnetic conditions (see Fig. 2). Thanks to these results, a vigorous debate on the spin diffusion length can be concluded and this new method will play an important role in the fields of quantum information and spintronics.
References
- [1] Y. Niimi, D. H. Wei, H. Idzuchi, T. Wakamura, T. Kato, and Y. Otani, Phys. Rev. Lett. 110, 016805 (2013).
- [2] L. Liu, R. A. Buhrman, and D. C. Ralph, arXiv:1111.3702.