Heat Capacity Measurement of Heavy Fermion Compound YbCo2Zn20 under Magnetic Field
Uwatoko Group
Recently, one of the most interesting topics has been the ground state properties of heavy fermion compounds located close to a magnetic Quantum Critical Point (QCP). Applying pressure or magnetic field controls the electronic configuration, and thus anomalous behaviors appear at around magnetic QCP, such as an unconventional superconductivity and non-Fermi liquid state. In the case of Yb based heavy fermion compounds, it is known that YbCo2Zn20 is a prototypical compound of the pressure-induced QCP from a paramagnetic state to an antiferromagnetic. The high temperature magnetic susceptibility shows the Curie-Weiss law with the effective moment close to the value of Yb3+ ( μeff = 4.5 μB), although there is no indication of magnetic order down to 20 mK. The low temperature electrical resistivity and specific heat follows ρ = ρ0 + AT2 and C/T = const : which indicates the Fermi-liquid behavior: the values of A and γ are 165 Ωcm/K2 and 7900 mJ/moleK2, respectively. These large values of A and γ are due to the small Kondo temperature, suggesting the closeness to the pressure induced magnetic order. According to the resistivity, specific heart and neutron diffraction measurements under pressure, magnetic ordered phase appears around critical pressure Pc = 1 GPa. Figure 1 shows the pressure phase diagram of YbCo2Zn20 [1]. The applying pressure stabilizes the magnetic Yb3+ configuration and resulting in the appearance of a magnetically ordered state. It is worth noting that the critical pressure of YbCo2Zn20 is lower than that of other Yb based compounds such as YbNi2Ge2 (Pc = 5 GPa) and YbCu2Si2 (Pc = 8 GPa). Therefore YbCo2Zn20 has an advantage of investigating the behavior of Yb based compounds near QCP.
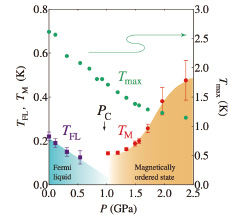
Fig. 1. Pressure–temperature phase diagram of YbCo2Zn20. Tmax and TFL are suppressed by applying pressure, and thus, the nonmagnetic Fermi-liquid state vanishes around Pc. TM appears to abru ptly develop above Pc, where Tmax decreases. Assuming that the observed Tmax in the resistivity is proportional to TK, the value of TK decreases with an increase in the pressure. According to a theoretical study, the suppression of valence fluctuations leads to a decrease in TK. It is generally accepted that the magnetic instability results from the competition between the RKKY and the Kondo interactions. By applying pressure, the RKKY interaction becomes more dominant with a reduction in the Kondo interaction.
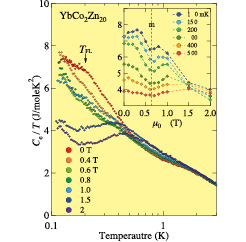
Fig. 2. Temperature dependence of the electronic contribution of the specific heat for YbCo2Zn20 at selected magnetic field (H // [110]). Arrow indicates the onset temperature of the Fermi liquid behavior. Here, the electronic contribution to the specific heat Ce of YbCo2Zn20 was obtained by subtracting that of LuCo2Zn20. At lower temperature, Ce/T increases logarithmically and tends to saturate below TFL ~ 0.2 K, coinciding with the T2 dependence in the resistivity, namely Fermi-liquid behavior as observed in the previous report. Inset : Field dependence of Ce/T at selected temperature. Two peak structure, which has local minimum point at Hm, was observed at around 0.6 T.
More interestingly, it is reported that a metamagnetic crossover occurs at Hm ~ 0.57 T by M. Ohya et al. The origin of this metamagnetic behavior is not fully understood, however relevance to the valence fluctuation is considered by S. Watanabe and K. Miyake. To shed light on the nature of the metamagnetic behavior, the specific heat of YbCo2Zn20 single crystal under magnetic field has been measured at ambient pressure. Figure 2 shows the temperature dependence of the electronic contribution of the specific heat Ce/T under magnetic field on a logarithmic temperature scale. At zero- field, Ce/T exhibits a broad shoulder structure at around 2 K due to the combined effect of the Kondo effect and the Schottky anomaly associated with the crystalline electronic field (CEF) splitting. With increasing magnetic field, the TFL is gradually suppressed to a lower temperature. It is worth noting that Ce/T diverges more rapidly than a logarithmic dependence at Hm ~ 0.6 T, which corresponds to the metamagnetic field. With further increasing magnetic field, the temperature dependence changes to a peak structure as typical behavior of Kondo systems under magnetic field. Ce/T as a function of magnetic field at selected temperature has been plotted to see the field variation in inset of fig. 2. The most intriguing feature obtained here is the two peak structure which has local minimum point at Hm ~ 0.6 T. Although the double peak structure becomes weaker with increasing temperature, the minimum point is temperature independent. Similar feature is also observed in the prototypical heavy fermion compound CeRu2Si2 which shows metamagnetic transition at Hm ~ 7.7 T by J. Flouquet et al. In the case of CeRu2Si2, the field dependence of Ce/T exhibits the double peak structure above 1 K, and thus that changes to a single peak structure at lower temperatures. Here we compare the characteristic energy scales of YbCo2Zn20 to those of CeRu2Si2. It is obvious that the values of Hm and Tmax of YbCo2Zn20 are ten times smaller than that of CeRu2Si2, i.e. the characteristic energy scale of YbCo2Zn20 is much smaller than that of CeRu2Si2. Considering the difference of the energy scale, it is expected that the double peak structure in YbCo2Zn20 may evolve into a single peak structure at lower temperature, suggesting the development of the critical fluctuation. Further experimental and theoretical studies are needed to clarify the origin of the metamagnetic behavior and the relationship with the pressure-induced magnetically ordered state of YbCo2Zn20.
References
- [1] Y. Saiga, K. Matsubayashi, T. Fujiwara, M. Kosaka, S. Katano, M. Hedo, T. Matsumoto and Y. Uwatoko, J. Phys. Soc. Jpn. 77, 053710 (2008).