Magnetic Impurities in Spin Nematic State
Tsunetsugu Group
Quantum spin systems with strong frustration provide a nice playground for the quest of novel quantum orders. The spin-1 antiferromagnet with stacked triangular-lattice structure NiGa2S4 discovered by Nakatsuji Group has attracted attention of many experimental and theoretical groups to its spin-liquid like properties. Several theories have been proposed for their explanation, and one of the earliest is a spin nematic scenario put forward by our group. This can explain the absence of magnetic long-range order, particularly neutron scattering data, and power-law temperature dependence of specific heat at low temperatures, at least in qualitative level. However, this theory seems inconsistent with the divergent behavior observed by Ishida group in NMR relaxation rate around T*~8.5K, which is usually attributed to some magnetic phase transition.
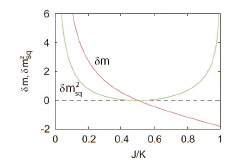
Fig. 1. Induced magnetic moments around impurity. δm is the total magnetization of induced moments, and δmsq2 is the sum of squared moments.
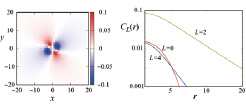
Fig. 2. Interactions between impurity moments mediated by nonmagnetic excitations in the host for J/K=0.5. Coordinates are measured in units of lattice constant, while the unit of interactions is K. (Right) Angular part decomposition of the interaction, ΣLCL(r)cosLφ.
We have tried to explain this magnetic “instability” within the spin nematic scenario. One useful hint is μSR experiment performed by MacLaughlin and his colleagues. They reported the presence of slow spin dynamics even below T* and its time scale is order of μs. Magnetic susceptibility also indicates a glassy behavior below T*, but its glassy signal is very weak. Another hint is an unconventional impurity effect. Upon doping impurities, the freezing temperature T* decreases, and this is opposite to the conventional case of canonical spin glasses where the freezing temperature is enhanced.
Our idea is that impurity magnetic moments establish unconventional type of interactions among them in the host with spin nematic order and that these interactions lead to a glass state of impurity moments with slow dynamics. The first program is to elucidate the nature of impurity interactions. This question is also interesting in that impurities may be used as useful probe to investigate host properties. A well-known example is RKKY interaction of magnetic impurities in metal, and it has two characteristics. One is a long-range nature; interaction decays in a power-law rather than exponential. The other is oscillation in space, and interaction changes from antiferromagnetic to ferromagnetic and vice versa with impurity distance. The first is a manifestation of the presence of zero-energy magnetic excitations in the host metal. The second is the consequence of the presence of a typical length scale, which is the inverse of the linear size of Fermi volume.
Our system has a characteristic that the host has a noncoplanar spin nematic order with a finite ordering wave vector. The spin nematic order has gapless nonmagnetic excitations as its Goldstone mode due to its spontaneous breaking of spin rotation symmetry. We have performed calculations in the sprit of RKKY interaction. We first calculate how a single magnetic impurity creates both nonmagnetic and magnetic excitations in its neighboring part of the host. To determine interaction between a pair of impurities, we then calculate the energy cost or gain due to overlap of these excitations created around each of the two impurities. The model is a spin-1 Hamiltonian with bilinear (J) and biquadratic (K) interactions of Ni-ion spins. This is a phenomenological model for investigating an antiferro spin nematic order, and the both J and K are antiferromagnetic. We have employed site-dependent mean-field approximation combined with analysis with effective field theory.
Solution of a single impurity problem provides two important results. The first point is that the host nematic order generates effective spin anisotropy of impurity moments. Details of anisotropy depend on the value of K/J, but in most of the parameter space considered (0.087<J/K<1), magnetic moments tend to move apart as much as possible from directors of the antiferro nematic order. In a narrow region near the boundary with the ferromagnetic phase (0< J/K <0.082), the easy axes are parallel to the directors instead. The second point is that there appear induced magnetic moments in a neighboring part of the host around the impurity. Figure 1 shows the total induced moment, and it is divergently enhanced near the border to ferromagnetic phase (J/K→0) or antiferromagnetic phase (J/K→1). This means that even when the impurity concentration is small, many magnetic moments are activated there. We have also calculated created nonmagnetic and magnetic excitations around the impurity. The results show that nonmagnetic one has a long tail in its spatial distribution, and this decay is proportional to 1/(distance). It also changes sign when one goes around the impurity site. Magnetic excitation is exponentially localized around the impurity, but its size is again divergently enhanced near the two borders, ξ ∝ (K/|K-2J|–1)–1/2.
We have calculated impurity-impurity interactions mediated by nonmagnetic and magnetic excitations around impurities. The dominant part is the contribution of nonmagnetic excitations, and its dependence on impurity-impurity distance is plotted in Figure 2. Decomposing it to its angular harmonics, we find that the L=2 mode is the largest component. This interaction changes its sign four times when one impurity moves around the other, and it has two important features. One is the long-range nature, i.e. dependence ~1/r2. The other is that this is an interaction of spin quadrupole moments rather than dipole moments: the impurity Hamiltonian is biquadratic in their magnetizations. Since the interactions are long-ranged and oscillating in space, they stabilize a glassy state. However, this state differs in nature from the ordinary spin glass state in that the frozen degrees of freedom are spin quadrupoles rather than dipoles. Conventional pair-wise spin flips do not cost this energy, and they persist in the glassy phase. We expect that this is the origin of slow spin dynamics observed in μSR measurements. Since this long-range interaction is mediated by low-energy excitations in the ordered host, the glassy state should disappear together, when the spin nematic order vanishes. Thus, the freezing temperature T* is determined by the bulk energy scale, and is suppressed by impurity doping. To provide quantitative predictions for the glassy state, we need further investigations, and we believe that this may be a good starting point.
References
- [1] J. Takano and H. Tsunetsugu, J. Phys. Soc. Jpn. 80, 094707 (2011).