Nonperturbative Conserving Approximation Scheme for Self-Consistent Calculation of the Electron Self-Energy
Takada Group
The electron self-energy Σ is a fundamental quantity in the Green's-function approach to the many-electron problem in solids. It is directly related to the one-electron spectral function A(k,ω) that can be observed experimentally by angle-resolved photoemission spectroscopy (ARPES). Accurate information on Σ(k,ω) in the wide range of momentum k and energy ω is useful not only for elucidating the detailed Fermi-liquid (FL) properties in usual metals but also for discussing the non-Fermi-liquid behavior inherent to the Tomonaga-Luttinger liquid (TLL) in systems with one spatial dimension.
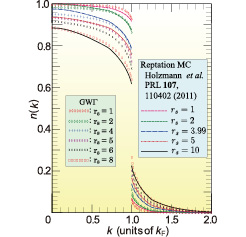
Fig. 1. Momentum distribution function n(k) for the electron liquid at rs=1 (diamond), 2 (ellipse), 4 (plus), 5 (star), 6 (cross), and 8 (rectangle) in the GWΓ method in comparison with those for rs=1 (longer dashed), 2 (dashed), 3.99 (dotted-dashed), 5 (dotted), and 10 (solid) in the reptation Monte Carlo method.
Usually, Σ is calculated with use of many-body perturbation-theoretic (MBPT) methods, such as the Hedin’s GW approximation (GWA). This GWA is a conserving approximation in the Baym-Kadanoff's sense, obeying the conservation laws of the macroscopic quantities like the total electron number, but because of the omission of all vertex corrections it does not satisfy the Ward identity (WI), an exact relation between Σ and the vertex function Γ due to gauge invariance representing the local electron-number conservation. Under the violation of the WI, an accurate enough Σ is not obtained in FL metals. In TLL metals, the situation becomes even worse, because any MBPT approach is inappropriate from the outset for describing the nonperturbative features of the TLL.
About a decade ago, a nonpertubative approach or the GWΓ scheme was developed for the self-consistent calculation of Σ[1], based on a rigorous algorithm to obtain the exact Σ as a fixed point of the self-energy revision operator [2]. This scheme always satisfies the WI and is applicable to both FL and TLL in a unified manner, but it encountered a serious problem in the electron liquid with the densities lower than the metallic ones; convergent results for Σ are not obtained, if its density specified by the dimensionless parameter rs is larger than 5.25 at which there appears the dielectric catastrophe associated with the divergence of the compressibility κ and concomitantly that of the static polarization function Π in the long wave-length limit.
In view of this situation, we have revised the original GWΓ scheme by (i) giving the better functional form for Γ based on a detailed analysis of the analytical structure of the exact Γ, (ii) modifying the scheme itself into the one free from the difficulty originating from the dielectric catastrophe, and (iii) proposing an alternative scheme on the condition that an accurate piece of information is available for Π by other calculations or experiments [3]. As an example to illustrate the power of our scheme, the results for the momentum distribution function n(k) are shown in Fig. 1 in the low-density electron gas for rs up to 8 in comparison with those recently obtained by the reptation Monte Carlo (RMC) method. In contrast to the inaccuracy in RMC, our results are so accurate that they all satisfy the important sum rules of Σkσk2nn(k) for n=0 (total number), n=1 (kinetic energy), and even n=2 (fluctuation of kinetic energy) at least up to six digits.
In the future, we shall apply this scheme to real solids, along with (i) pursuing further analysis of the emergence of electron-hole asymmetric excitations, leading to a very characteristic n(k) and a self-induced excitonic state for rs > 8, and (ii) exhibiting transmutation from bosonic to fermionic natures with the increase of excitation energies in the one-dimensional TLL.
References
- [1] Y. Takada, Phys. Rev. Lett. 87, 226402 (2001).
- [2] Y. Takada, Phys. Rev. B 52, 12708 (1995).
- [3] H. Maebashi and Y. Takada, Phys. Rev. B 84, 245134 (2011).