Superconducting Gap Structure of CeIrIn5 from Field-Angle-Resolved Measurements of its Specific Heat
Sakakibara Group
The heavy-fermion systems CeMIn5 (M = Co, Rh, and Ir) have been extensively studied because they exhibit unconventional superconductivity near the antiferromagnetic (AF) quantum critical point. Recently, field-angle-resolved specific heat C(ø) experiments along with the theoretical works have confirmed that CeCoIn5 is a dx2-y2 -wave superconductor [1], and its pairing interaction is widely accepted to be AF spin fluctuations. Similarly, from the C(ø) experiment, pressure induced superconductivity in CeRhIn5 is argued to have the same pairing symmetry [2]. By contrast, the gap structure of CeIrIn5 (Tc = 0.4 K) has remained controversial. While the dx2-y2 -wave gap was supported from the field-angle-resolved thermal conductivity measurements [3], the gap function kz or kz(kx+iky), both of which have a horizontal line node only on the equator and are in sharp contrast to the dx2-y2 -wave gap, was suggested from the effect of impurity scattering on the thermal conductivity [4]. In order to settle the controversy over the gap structure of CeIrIn5, we have performed C(ø) measurements down to 80 mK [5].
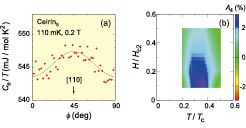
Fig. 1. (a) Variations of the specific heat as a function of the azimuthal angle ø between the [100] axis and the magnetic field applied in the ab plane. (b) A contour plot of the normalized fourfold amplitude A4(T, H) of CeIrIn5.
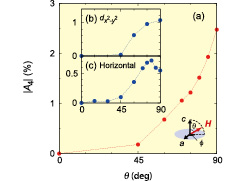
Fig. 2. (a) Polar angle θ dependence of A4 obtained by conically rotating magnetic field with the strength of 0.2Hc2(θ) at 110 mK. Calculated θ dependence of A4 for (b) the dx2-y2-wave gap and (c) the horizontal line node gap. The observed monotonic variation of A4 with θ confirms the absence of a horizontal line node on the equator and strongly supports the dx2-y2-wave gap.
Figure 1(a) shows the specific heat measured in magnetic field H rotated in the tetragonal ab plane. A clear fourfold oscillation was observed with maxima in fields along the <110> direction at 110 mK and 0.2 T. To characterize the H and T variations of the fourfold oscillation, we fit the data to the expression C0(T) + CH(T, H)(1 - A4cos4ø) and show the contour plot of A4(T, H) in Fig. 1(b). Here, C0 and CH are the zero-field and field-dependent components of the electronic specific heat, respectively, and A4 is the amplitude of the fourfold oscillation normalized by CH . We found that A4(T, H) exhibits a peak at around 0.3Tc and 0.15Hc2, and rapidly decreases down to zero around 0.2Tc. This observed feature can be well reproduced by the microscopical calculations assuming the dx2-y2 -wave gap.
However, as claimed in Ref. [4], the ø rotation experiment alone cannot rule out the possibility of the horizontal line node. In order to solve this issue, we investigated C(ø) by conically rotating H at several fixed θ, where θ denotes the polar angle between H and the c axis. As shown in Fig. 2(a), A4 is monotonically suppressed by tilting H out of the ab plane. According to the microscopical calculations, a gradual and monotonic decrease of A4 on decreasing θ from 90 deg is expected for the dx2-y2 -wave gap (Fig. 2(b)), whereas A4(θ) is predicted to have a dip around θ =90 deg for the horizontal line node gap (Fig. 2(c)). It is obvious that the dx2-y2 gap rather than the horizontal line node gap better reproduces the experimental data. In particular, the absence of the dip of A4(θ) at θ = 90 deg confirms the absence of a horizontal line node on the equator. These observations lead us to conclude that the superconducting gap in CeIrIn5 is of dx2-y2 type gap, as in CeCoIn5 and CeRhIn5. The establishment of the identical gap symmetry in CeMIn5 (M = Co, Rh, and Ir) indicates the universality of the pairing mechanism in this family and provides important hints for resolving the mechanism of the novel superconductivity.
References
- [1] K. An et al., Phys. Rev. Lett. 104, 037002 (2010).
- [2] T. Park, E. D. Bauer, and J. D. Thompson, Phys. Rev. Lett. 101, 177002 (2008).
- [3] Y. Kasahara et al., Phys. Rev. Lett. 100, 207003 (2008).
- [4] H. Shakeripour et al., Phys. Rev. B 82, 184531 (2010).
- [5] S. Kittaka et al., Phys. Rev. B 85, 060505(R) (2012).