Spin-Orbital Short-Range Order in the Honeycomb Based Lattice of Ba3CuSb2O9
Nakatsuji Group
Nature favors the lowest energy so called ground state of a system, but at high temperatures disorder, called entropy, is dominant and provides the driving force for fascinating behavior such as phase transition from solid to liquid and from liquid to gas. Conversely, when temperature is lowered, the system solidifies because atoms or molecules, which move relatively freely in gas or liquid phase, spontaneously break the symmetry of the system to reduce its energy, leading to variety of intriguing phenomena. However, in some cases the liquid state can survive down to lowest temperature by lowering its quantum mechanical energy, which is known as quantum liquid. Examples are the superfluidity of Helium and the superconductivity of electrons. These quantum states have attracted much attention not only as a scientific subject of importance, but also as a subject in an applied research because of its robustness against disorder and the absence of dissipation at the quantum level.
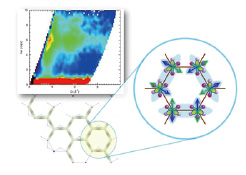
Fig. 1. Top left: Energy gap of spin liquid state revealed by inelastic neutron scattering experiments at 25 K. Bottom left: Short-ranged honeycomb-based lattice of Ba3CuSb2O9 Right: Spin-orbital quantum state on the honeycomb-based lattice. Neighboring orbitals (green) forms a ferro-order. Arrows (blue and green) and blue shaded regions indicate spin-singlet dimers having a spin gap.
Recent studies on magnets revealed that, under particular circumstances, the spins and orbitals which carry magnetism can remain liquid down to the lowest temperature with unbroken symmetry. This unusual behavior has attracted much attention because of its possibility as a new quantum liquid. It has been recognized, however, that such a liquid state of spin or orbital character is fragile and is easily frozen by, for example, structural disorder in magnets. In the present study on a copper oxide, we have discovered a new quantum liquid state that is robust against disorder by controlling cooperative phenomenon of spins and orbitals in electrons of copper atoms [1].
The material is Ba3CuSb2O9, consisting of electric dipoles formed by a pair of divalent copper ions (Cu) and pentavalent antimony ions (Sb). This material was previously considered to be a ferroelectric in which the electric dipoles form a triangular lattice [2,3]. However, our recent diffraction measurements using synchrotron x-ray at SPring-8 revealed that the intrinsic aspect of this material is frustration on triangular lattice, and, as a result, the electric dipoles spontaneously form a short range ordering, which intrisically stabilizes the disordered honeycomb lattice of CuO6 octahedra against the Jahn-Teller distortion.
Generally, cations with orbital degrees of freedom exhibit orbital order by lowering the symmetry of the spatial distribution of surrounding anions (ligands) so that the electrostatic energy of each orbital is reduced. This phenomenon is called “Jahn-Teller phase transition”. The typical example of this phenomena are copper oxide based materials famous for high-temperature superconductivity, and it is known that all copper oxide based materials have a Jahn-Teller phase transition.
In this work we have performed a comprehensive study of structural and magnetic properties of Ba3CuSb2O9 using high-quality single crystal samples, and discovered that this material shows neither magnetic ordering nor a macroscopic Jahn-Teller phase transition down to low temperature. In addition, neutron scattering measurements (US-Japan Cooperative Program) revealed that copper ions form a dimer, a local resonant state of S = 1/2 of a copper ion and a neighboring copper ion (Fig. 1). On the other hand, X-ray absorption fine structure measurements found that all copper ion sites undergo Jahn-Teller distortion for short time scale as well as for local length scale. These observations indicate two possibilities: firstly, the orbitals order with a ring pattern along the honeycomb hexagon so that the spins can form the pair of the local spin dimer and secondly, both spins and orbitals cooperatively form a dynamic state which is a quantum mechanical resonant state (Fig. 1).
The realization of state without macroscopic Jahn-Teller phase transition in copper oxides is quite remarkable, and this state may stabilize a spin liquid, a novel quantum mechanical state. Furthermore, this state originates from a self-assembly of copper ions due to the electrostatic interaction between electric dipoles made by copper and antimony. This indicates that the quantum properties in condensed matter can be controlled using the pattern of electric dipoles, and provides an importance guide for future materials development. The present discovery of a material that shows quantum liquid state robust against disorder can open a new route to exploring materials required for the practical development of quantum information processing and quantum computing.
References
- [1] S. Nakatsuji, K. Kuga, K. Kimura, R. Satake, N. Katayama, E. Nishibori, H. Sawa, R. Ishii, M. Hagiwara, F. Bridges, T. U. Ito, W. Higemoto, Y. Karaki, M. Halim, A. A. Nugroho, J. A. Rodriguez-Rivera, M. A. Green, and C. Broholm, Science 336, 559 (2012).
- [2] V. P. Köhl, Z. anorg. allg. Chem. 442, 280 (1978).
- [3] H. D. Zhou, E. S. Choi, G. Li1, L. Balicas, C. R. Wiebe, Y. Qiu, J. R. D. Copley, and J. S. Gardner, Phys. Rev. Lett. 106, 147204 (2011).