Ferroelectricity in Magnetite
Lippmaa Group
Magnetite Fe3O4 is among the oldest known naturally-occurring magnetic materials. The ferrimagnetic order in magnetite is derived from unequal numbers of spins in two antiparallel Fe sublattices divided among the tetrahedral and octahedral sites of the spinel crystal structure. Magnetite is metallic at room temperature, but a charge-ordered state appears when a crystal is cooled below the Verwey temperature of about 125 K, resulting in a sharp increase of resistivity. The charge-ordered state has been predicted to be polar and ferroelectric, but accurate measurement of ferroelectric polarization is difficult due to the relatively low resistivity of magnetite just below the Verwey transition temperature.
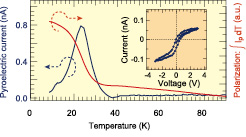
Fig. 1. Temperature dependence of the measured pyroelectric current amplitude under periodic heating of a thin film Fe3O4 sample capacitor. The measured current can be integrated to obtain a polarization curve. By also applying periodic poling bias pulses, the ferroelectric polarization loop can be measured (inset).
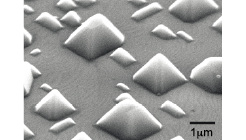
Fig. 2. Scanning electron microscope image of nanoscale magnetite pyramids with a (001) lattice orientation grown by a self-template technique on a (001) SrTiO3 substrate.
A number of zero-bias techniques can be used to accurately measure the ferroelectric polarization, even in materials that are not insulators. We have chosen to use a pyroelectric technique, where a miniature thin-film capacitor is periodically heated with a focused laser beam. Since the polarization of ferroelectrics is temperature dependent, it is possible to measure the derivative of the polarization-temperature curve by integrating the current flowing in or out of the sample capacitor during periodic heating and cooling. Since no bias is applied to the capacitor during measurement, characterization for ferroelectric polarization is not affected by leak currents. Figure 1 shows an example of a pyroelectric polarization measurement for a thin magnetite film. Integrating the pyroelectric current is used to obtain the polarization curve, while a ferroelectric hysteresis curve is obtained by poling the ferroelectric capacitor with short applied bias pulses and varying the applied pulse amplitude between -3 and +3 Volts. It is clear that the magnetite film shows spontaneous polarization below about 40 K and the polarization direction can be switched by applied bias.
Ferroelectricity in oxides is usually related to the freezing of a soft mode that results in a structural phase transition. Magnetite is an interesting case, since Raman spectroscopy measurements show no structural transitions in magnetite films below the Verwey temperature. The appearance of ferroelectricity can therefore be assumed to be caused by a purely electronic mechanism, related to bond dimerization in the charge-ordered state. In order to further study the ferroelectric transition in magnetite, it is important to obtain films with a well-defined crystal orientation and to avoid the appearance of a random multidomain state. We have developed a self-template thin film growth technique for fabricating such films on (001)-oriented SrTiO3 single-crystal substrates. The technique is based on higher-order lattice matching that allows the much larger Fe3O4 spinel lattice to form an epitaxial film on a perovskite SrTiO3 substrate. The higher-order lattice-matched magnetite buffer layer must be grown at low temperatures to stabilize the thermodynamically unfavorable (001) film surface. Once the buffer has been grown, a thick magnetite film can be grown at high temperature without losing the desired (001) lattice orientation. Very high-quality films can be grown even at 1100 °C, where surface faceting leads to the formation of nanoscale pyramids (Fig. 2), while still maintaining the (001) lattice orientation. We expect such nanopyramids to be good candidates for obtaining small single-domain magnetite crystallites for pyroelectric characterization of the ferroelectric state in Fe3O4.
References
- [1] R. Takahashi, H. Misumi, and M. Lippmaa, Cryst. Growth and Design 12, 2679 (2012).