Vortex State in hcp solid 4He
Kubota Group
Superfluidity has been found not only in the liquid state of two isotopes of He, but also in electrons (superconductivity) in solids as well as in dilute gas systems. As to much higher density systems, possible superfluidity in the solid state had been discussed since early days from 1956 by Penrose and Onsagar with negative result [1]. Positive theoretical discussions for the superfluidity in solids were strengthened in 1960’s and quite positive expectations were expressed by various groups in the world in 1969 and 1970[2]. Since then a lot of experimental efforts to find it out took place over the world including Japanese groups. It is, however, 2004 when the first convincing evidence was presented as a possible observation of the non-classical rotational inertia (NCLI) in solid He[3]. 8 years have passed since then yet the real happening in the solid He is still controversial [4].
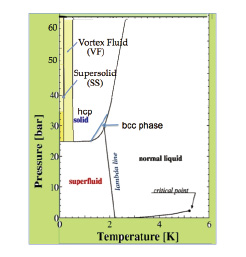
Fig. 1. P-T phase diagram of 4He deduced from the TO measurements. The vortex fluid (VF) state continues to T = 0K from the onset temperature To ~ 500 mK, while the supersolid (SS) state appears below Tc =~75 mK.
The Kubota group had been developing a technique to study essential features of superfluids in an superfluid made of 3D connected He monolayer superfluid film[5] using a technique combining highly sensitive torsional oscillator (TO) detection and DC rotation of the sample (whole cryostat) at very low temperatures. TO measures the moment of inertia, through which change of momentum of the bob where the sample is located is detected. For a superfluid non-classical rotational inertia NCRI is measured as a decrease of the moment of inertia. More generally the decrease of the moment of inertia is expected for a vortex fluid state and the TO response is called nonlinear rotational susceptibility NLRS after Anderson [6]. TO measures also the Q value change through which the energy dissipation in the system is measured. DC rotation of the superfluid sample would cause vortex lines through the superfluid/supersolid. They [5,7,9] discovered a new way of detecting quantized vortex lines through the sample utilizing the fact that the circulating superflow around each of the vortex lines penetrating through the sample interacts with quasi particles in the system and causes extra energy dissipation [5]. Highly sensitive torsional oscillator technique has been employed for the study of solid He as well under DC rotation [7] to detect the extra dissipation below a transition temperature Tc, below which macroscopic coherence is expected to be established in the system. Evidence for the vortex lines penetration was found. In addition they found the occurrence of the hysteretic behavior just below the same Tc and argued that one branch of the hysteretic loop expels the vortices from the sample and the difference between the two branches of the NLRS gives the supersolid density ρss. Rather complicated situation in the study of the vortex state and the supersolid state in hcp 4He is clarified in the recent review paper by Minoru Kubota[8] from the independent different kinds of quantitative measurements on the same samples. Namely the supersolid density ρss is derived from the hysteretic component of the NLRS and the energy dissipation caused by introduction of vortex lines through the sample in one of the systeretic state, equilibrium state where vortices are penetrated through the sample, while the excited state rejects external vortices.
Hysteretic behavior is known for some of the type II superconductors as the de Gennes, Bean & Lipingston effect [9], where surface barrier makes the sample diamagnetic just in the similar manner as in the Meissner state.
References
- [1] O. Penrose and L. Onsager, Phys. Rev. 104, 576 (1956).
- [2] G. V. Chester, Phys. Rev. A 2, 256 (1970); A. F. Andreev and I. M. Lifshitz, JETP 29, 1107 (1969); A. J. Leggett, Phys. Rev. Lett. 25, 1543 (1970).
- [3] E. Kim & M. H. W. Chan, NATURE 427, 225 (2004); Science 305, 1941 (2004).
- [4] See for the reviews till 2007 or 2008; N. Prokof’ev, Adv. Phys. 56(2), 381 (2007); S. Balibar and F. Caupin, J. Phys.: Condens. Matter 20, 173201 (2008); D. Galli and L. Reatto, J. Phys. Soc. Jpn. 77(11), 111010 (2008).
- [5] M. Fukuda, M.K. Zalalutdinov, V. Kovacik, T. Minoguchi, T. Obata, M. Kubota, and E. B. Sonin, Phys. Rev. B 71, 212502 (2005).
- [6] P. W. Anderson, Nature Physics 3, 160 (2007)
- [7] M. Yagi, A. Kitamura, N. Shimizu, Y. Yasuta, and M. Kubota, J. Low Temp. Phys. 162, 492 (2011); ibid, 162, 754.
- [8] M. Kubota, submitted to J Low Temp Phys, special issue on solid He (2012); arXiv:1203.6824. see also references in it.
- [9] De Gennes, "Superconductivity in Metals and Alloys”, Benjamin, (Frontiers in physics series); Lecture notes from a course given in the Faculty sciences, Orsay, France, 1962-63, 76 (1965); C.P. Bean and J.D. Livingston, Phys. Rev. Lett. 12, 14 (1964).