Geometric Resonances in Hexagonal Lateral Superlattices – An Initial Step towards Artificial Graphene
Iye Group
A hexagonal lateral superlattice (LSL) – a two-dimensional electron gas (2DEG) subjected to periodic potential modulation having hexagonal-lattice symmetry – is of interest in two different directions. First, it is envisaged as a route to artificially generate massless Dirac fermions at the corners of the superlattice Brillouin zone [1]. Second, it is expected to stabilize the fragile “bubble phase” in the quantum Hall system (clusters of two or three electrons arranged in the hexagonal lattice, theoretically predicted to be the ground state at the 1/4 or 3/4 filling of the third or higher Landau levels [2]). As an initial step towards experimental verification of these fascinating possibilities, we study magnetotransport of hexagonal LSLs. We find three types of oscillations that evidence the hexagonal-lattice potential imposed on the 2DEGs [3].
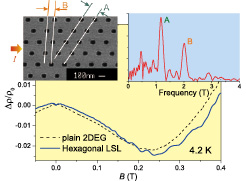
Fig. 1. Magnetoresistance Δρ/ρ0 of the hexagonal LSL (a = 200 nm) showing commensurability oscillations resulting from the periodicities A and B. Magnetoresistance of the plain 2DEG is also shown for reference. Left inset: Scanning electron micrograph of the hexagonal array of the EB resist (black dots). Periodicities A and B are indicated. Right inset: Fourier spectrum of the oscillatory part (d2/dB2)(Δρ/ρ0) vs. 1/B.
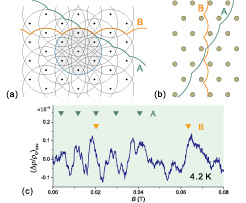
Fig. 2. (a) Hexagonal lattice in the reciprocal space, showing Fermi circles and open orbits originating from Bragg reflections due to periodicities A and B. (b) Open orbits in the real space. (c) Oscillatory part of the low-field magnetoresistance (obtained by subtracting slowly-varying background) of the hexagonal LSL (a = 200 nm). Downward triangles mark the magnetic fields for the geometric resonances of the open orbits A and B to take place.
The LSL devices are fabricated from conventional GaAs/AlGaAs 2DEGs. Periodic potential modulation is introduced via strain-induced piezoelectric effect by placing a hexagonal array of electron-beam (EB) resist pillars, with the lattice constant a = 100 nm or 200 nm, on the surface of the wafer (Fig. 1, left inset). The main panel of Fig. 1 shows magnetoresistance traces of a hexagonal LSL with a = 200 nm at 4.2 K. Oscillations are observed above ~ 0.1 T. Fourier analysis shown in the right inset reveals that the oscillations are composed of two 1/B-periodic components, attributable to the commensurability between the periodicity A (173 nm) and B (100 nm), respectively, depicted in the left inset, and the cyclotron radius Rc. At lower temperatures (~ 15 mK), Shubnikov-de Hass (SdH) oscillations are clearly resolved. In hexagonal LSLs, SdH oscillations exhibit amplitude modulation analogous to those observed in unidirectional LSLs [4]. The modulation can be explained by the commensurability oscillations of the Landau bandwidth due to the periodicity A, with the amplitude maxima taking place at the flatband conditions.
Closer inspection of lower magnetic field regime (B < ~ 0.1 T) divulges yet another type of oscillations having very small amplitudes, as displayed in Fig. 2 (c). A series of small peaks are ascribed to the occurrence of the geometric resonance of open orbits [5] composed of the segments of cyclotron orbits repeatedly diffracted by Bragg reflections (see Fig. 2 (a) and (b)). The width of the open orbits varies as 1/B, and the resonance takes place at the magnetic field where the width coincides with the corresponding periodicity. The observation of these resonances demonstrates that the miniband structure brought about by the externally imposed hexagonal superlattice can be explored by the measurement of the magnetoresistance, and allows us to envision the possibility of observing the Dirac points by further decreasing the lattice constant and/or increasing the Fermi wavelength.
References
- [1] C-H. Park and S. G. Louie, Nano Lett. 9, 1793 (2009).
- [2] A. A. Koulakov, M. M. Fogler, and B. I. Shklovskii, Phys. Rev. Lett. 76, 499 (1996).
- [3] Y. Kato, A. Endo, S. Katsumoto, and Y. Iye, Proc. 19th Int. Conf. on Electronic Properties of Two-Dimensional Systems (2011).
- [4] A. Endo and Y. Iye, J. Phys. Soc. Jpn. 77, 054709 (2008).
- [5] A. Endo and Y. Iye, Phys. Rev. B 71, 081303(R) (2005).