Observation of Strong Magnetoelectric Coupling in Multiferroic BiFeO3 above Room Temperature
Tokunaga Group
Recently, ferroelectric magnets, i.e. so-called multiferroic materials, have attracted considerable attention due to their richness of scientific interests and also potential application for novel memory devices with reduced power consumption. In this class of materials, most of the attractive phenomena are caused by the presence of strong coupling between the magnetic moment and the electric polarization (P). In general, the coupling between them cannot be strong because these two order parameters have different symmetry against time reversal and space inversion operation, and hence, do not have a linear coupling term in the expression of the free energy. This constraint is removed in some kinds of magnets with spatially modulated spin structures (SMSSs), in which spatial inversion symmetry is broken by the magnetic order [1,2]. Since such SMSSs usually originate from geometrical frustration in the spin system, the strong magnetoelectric coupling emerges only at low temperatures. In this context, BiFeO3 is the unique example that shows multiferroic nature above room temperature with large electric polarization of P ∼ 100 μC/cm2 [3,4].
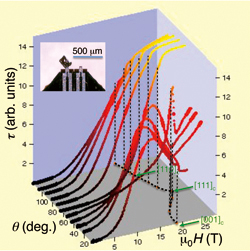
Fig.1. Magnetic field dependence of magnetic torque (τ) measured with using a micro-piezoresistive cantilever in various field directions at T = >4.2 K. The field direction was changed from the [001] to the [110] axis in the cubic notation. The transition field becomes the highest when the field is applied along the [111] direction. The thick dotted line schematically shows the calculated transition field based on the Ginzburg-Landau theory. The coincidence with the experimental results indicates the validity of this argument.
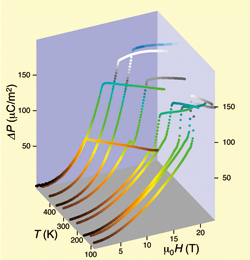
Fig.2 . Magnetic field-induced changes in P (ΔP) projected to the cubic [001] direction at various temperatures from 77 K to 500 K. Magnetic fields were applied perpendicular to the projection axis. The transition field becomes smaller with increasing the temperature toward the Néel temperature of ∼ 600 K. The field-induced change in P remains larger than 100 μC/m2 up to 450 K.
We studied the effect of magnetic fields on BiFeO3 using single domain crystals grown by the flux method [5]. At low temperatures, metamagnetic transitions accompanied with steep changes in P take place at magnetic fields of ∼ 19 T along the [100] direction of the pseudocubic lattice. Magnetic torque measurements in various field-directions show that the transition field becomes the highest when the magnetic field is applied along the [111] direction (Fig. 1). This angular dependence is reproduced by a phenomenological argument based on the Ginzburg-Landau theory, which indicates this transition to be a magnetic one from the cycloidal to the canted antiferromagnetic state. According to the recent microscopic theory [1], this change in the spin structure is accompanied with the disappearance of the parasitic P caused by the SMSS.
We studied temperature dependence of this parasitic component through the measurements of the field-induced change in P (ΔP) up to 500 K (Fig. 2). The transition field decreases with increasing temperature toward the Néel temperature of ~ 600 K, whereas the magnitude of the ΔP remains greater than 100 μC/m2 up to 450 K. Although this value is much smaller than that of the spontaneous polarization, it is comparable to those in other multiferroic materials. In particular, the evaluated microscopic coupling constant between the spin and electric polarization is even larger than that in the standard multiferroic material of TbMnO3. The present finding of the strong magnetoelectric coupling in BiFeO3 provides a strategy to design novel multiferroic materials of practical use.
References
- H. Katsura, N. Nagaosa, and A. V. Balatsky, Phys. Rev. Lett. 95, 057205 (2005).
- M. Mostovoy, Phys. Rev. Lett. 96, 067601 (2006).
- V. V. Shvartsman et al., Appl. Phys. Lett. 90, 172115 (2007).
- D. Lebeugle et al., Appl. Phys. Lett. 91, 022907 (2007).
- M. Tokunaga et al., J. Phys. Soc. Jpn. 79, 064713 (2010).