Incommensurate Order and Rotational-Symmetry Breaking in 2D Frustrated Heisenberg Model
Kawashima Group
The magnetic compound NiGa2S4 is a rare example of a two-dimensional triangular lattice antiferromagnet and stays in a spin-disordered state at low temperatures. Interesting behaviors of this material were observed by Nakatsuji group[1,2]. For example, development of incommensurate (IC) spin correlation was confirmed by magnetic neutron scattering experiment. It is easy to see that the incommensurate magentic ordering can be stabilized at least for zero-temperature by introducing a dominant antiferromagnetic third-nearest-neighbor interaction.
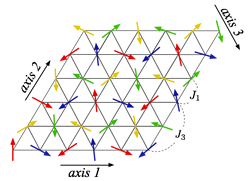
Fig.1. A schematic illustration of spin configuration for the ground state. The spin configuration along axis 1 is characterized by the wave number k, and that of axes 2 and 3 by k/2. There is a threefold degeneracy corresponding to 120 degree lattice rotations. The spin configuration on each of the four sublattices, represented by different colors, is close to, but not exactly, the 120 degree structure.
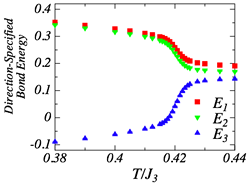
Fig. 2. Temperature dependence of the direction-specified bond energy E1, E2, and E3. The first-order transition temperature occurs Tc/J3 ~ 0.42.
Introduction of such intermediate-range interaction may cause various effects in addition to the incommensurate ordering. To study the consequence in more depth, we investigate a two-dimensional classical Heisenberg model on a triangular lattice with a ferromagnetic nearest-neighbor (NN) interaction J1 (<0) and an antiferromagnetic third-NN interaction J3 (>0). The model Hamiltonian is given by
If the third-NN interaction is dominant, there are three equivalent IC ground-state spin-configurations that can be transformed to each other by 120 degree lattice rotations (Fig. 1). Presence of this discrete symmetry makes it possible to have a finite temperature phase transition without violating Mermin and Wagner's theorem.
We perform classical Monte Carlo simulation using the standard heat-bath method[3]. In this calculation, the value of the interaction ratio J3/J1 is fixed at -3. We find that the specific heat as a function of the temperature exhibits a single peak indicating a phase transition. We study the probability distribution of the energy in order to determine the order of this transition. The distribution exhibits the bimodal structure and the valley in the middle of the distribution deepens with increasing lattice size. These evidences clearly suggest the first-order phase transition.
From ground state properties of the present model, it is natural to expect the spontaneous breaking of the threefold lattice-rotational symmetry. Based on this expectation, we calculate the direction-specified nearest-neighbor correlation along the three axes separately. To be specific,
In the symmetry breaking phase, one of the three εμ is negative, while the others are positive. We therefore sort the averages of the three quantities in descending order and define them as E1, E2, and E3. As shown in Fig. 2, E1 and E2 increase but E3 decreases below the first-order phase transition temperature. The threefold symmetry is spontaneously broken and one of the three axes is selected.
We now consider implications of these results on the experimental observations of NiGa2S4. The important point there was that the IC phase emerges at low temperatures and the specific heat has a broad double-peak structure. The present results are consistent with the incommensurability observed in the neutron scattering experiments. On the other hand, however, the specific heat of the present model only exhibits a single divergent peak. To relate the present results to the experiment, it is necessary to construct the whole phase diagram and also take into account other types of interactions that have been neglected.
References
- S. Nakatsuji, Y. Nambu, H. Tonomura, O. Sakai, S. Jonas, C. Broholm, H. Tsunetsugu, Y. Qiu, and Y. Maeno, Science 309, 1697 (2005).
- Y. Nambu, S. Nakatsuji, and Y. Maeno, J. Phys. Soc. Jpn. 75, 043711 (2006).
- R. Tamura and N. Kawashima, J. Phys. Soc. Jpn. 77 103002 (2008).