Determination of Element-Specific Complex Permittivity with Soft X-Ray Segmented Cross Undulator at SPring-8 BL07LSU
I. Matsuda, Akai, Wadati, Harada, and Shin Groups
Permittivity (or conductivity) is a quantity that characterizes a response of a matter by an electric field, such as an electromagnetic wave. It has historically been used to develop varieties of electronic and optical devices that have supported our society today. Determinations and understandings of permittivity of matters have been one of the highest priority tasks of science and technology. Recently, the requirement has reached to the ultraviolet (UV) ~soft X-ray (SX) region due to necessity of developing the extended-UV (EUV) lithography for the advanced technologies or ultrahigh-resolution SX experiments for the frontier sciences, for examples.
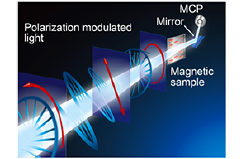
Fig. 1. Image of the polarization modulated light with the L-MOKE set up at SPring-8 BL07LSU.
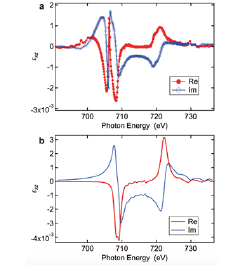
Fig. 2. a. εxz spectra of the Fe nanofilm at the L-edge obtained by the L-MOKE measurement with the polarization modulated light. Red lled circles and blue open circles represent its real and imaginary parts, respectively. b. εxz spectra of bulk Fe (bcc) at the L-edge obtained by the first-principles calculation with the KKR formalism. Red and blue lines represent its real and imaginary parts, respectively.
A permittivity has been often approximated as a (dielectric) constant but it is essentially the tensor quantity that is composed of diagonal and off-diagonal components. The diagonal and the off-diagonal element carries non-magnetic and magnetic information of a matter, respectively, and it is the latter that has been significant to understand the spin polarization or the magnetization. The individual elements are complex, being composed of the real and imaginary parts. The determination has been widely made by a probing light with the polarization modulation (frequency of p) and the measurement of the sample optical responses at the two frequencies (p, 2p). Such an experiment is easily achieved by transmitting the incident light through crystals that allow electric or mechanic controls of the polarization. Nowadays, one can find varieties of commercial polarization modulators for visible light and hard X-ray but for the EUV SX region. This is mainly because the light absorbance in a matter in this region is so large that it does not allow the transmission and the light can only survive in vacuum due to absorption by air molecules. In addition, the 'abnormal' optical response at the absorption edges of the composing element has also intervened the precise evaluation. Up to now, the determination has been made only with a help of simulations based on the atomic parameters and the indirect experimental data, which has often left the large uncertainty in the permittivity values. Thus, the estimation has been made for systems that are simple and not for the complicated or non-uniform ones. To overcome these difficulties, one can use synchrotron radiation, generated by electrons that already exist in vacuum and one can control the polarization by the electron trajectory that is regulated by the external magnetic field.
Here, we developed a novel SX source of the segmented cross undulator that can make the continuous polarization modulation (p) of the light and carried out the magneto-optical measurement (p, 2p) of the buried Fe nanolm at the Fe L-edge absorption edge in SX region (Fig. 1). As shown in Fig. 2, we succeeded in directly determining the complex permittivity and we found that the first-principles calculation with the Korringa-Kohn-Rostoker (KKR) formalism gave the perfectly matching results. It is of note that a spectral dip/peak at 705 eV in experiment is due to the optical response by the film structure that has not been taken into account in the calculation. Our procedure determines the permittivity of a sample in UV~SX region with high precisions, which is significant for the better understanding of the light-matter interaction. It also opens new technical examination method to seek for the best materials for optical elements, for examples, in the EUV lithography technology or in the laser technology of high harmonic generation lasers, high-brilliant synchrotron radiation, and X-ray free electron laser.
References
- [1] S. Yamamoto, Y. Senba, T. Tanaka, H. Ohashi, T. Hirono, H. Kimura, M. Fujisawa, J. Miyawaki, A. Harasawa, T. Seike, S. Takahashi, N. Nariyama, T. Matsushita, M. Takeuchi, T. Ohata, Y. Furukawa, K. Takeshita, S. Goto, Y. Harada, S. Shin, H. Kitamura, A. Kakizaki, M. Oshima, and I. Matsuda, J. Syn. Rad. 21, 352 (2014).
- [2] I. Matsuda, A. Kuroda, J. Miyawaki, Y. Kosegawa, S. Yamamoto, T. Seike, T. Bizen, Y. Harada, T. Tanaka, and H. Kitamura, Nucl. Instrum. Methods Phys., Sect. A 767, 296 (2014).
- [3] Y. Kubota, Sh. Yamamoto, T. Someya, Y. Hirata, K. Takubo, M. Araki, M. Fujisawa, K. Yamamoto, Y. Yokoyama, M. Taguchi, S. Yamamoto, M. Tsunoda, H. Wadati, S. Shin, and I. Matsuda, J. Elec. Spec. Rel. Phenom. 220, 17 (2017).
- [4] Y. Kubota, M. Taguchi, Sh. Yamamoto, T. Someya, Y. Hirata, K. Takubo, M. Araki, M. Fujisawa, H. Narita, K. Yamamoto, Y. Yokoyama, S. Yamamoto, M. Tsunoda, H. Wadati, S. Shin, and I. Matsuda, Phys. Rev. B 96, 134432 (2017).
- [5] Y. Kubota, Y. Hirata, J. Miyawaki, S. Yamamoto, H. Akai, R. Hobara, Sh. Yamamoto, K. Yamamoto, T. Someya, K. Takubo, Y. Yokoyama, M. Araki, M. Taguchi, Y. Harada, H. Wadati, M. Tsunoda, R. Kinjo, A. Kagamihata, T. Seike, M. Takeuchi, T. Tanaka, S. Shin, and I. Matsuda, Phys. Rev. B 96, 214417 (2017).