Maximizing Tc by Tuning Nematicity and Magnetism in FeSe1-xSx Superconductors
K. Matsuura, Y. Uwatoko, and T. Shibauchi
In iron pnictides, high-Tc superconductivity appears near the antiferromagnetic phase, which is accompanied by the tetragonal-to-orthorhombic structural transition with significant electronic anisotropy (nematicity). It has been discussed that the quantum fluctuations of nematic and/or antiferromagnetic orders may play an essential role for the superconductivity. However, these two orders coexist with each other in iron-pnictide superconductors, and thus it has been difficult to disentangle the role of each phase for the superconductivity. To address this issue, FeSe is an important material because it shows a non-magnetic nematic order below 90 K [1]. Moreover, by isovalent S-substitution which corresponds to the chemical pressurization, this order is suppressed with increasing the S-content and it has been found that a non-magnetic nematic quantum critical point exists in the phase diagram of FeSe1-xSx [2]. On the other hand, the non-magnetic nematic phase is also suppressed under physical pressure in FeSe, but before reaching a quantum critical point, a dome-shaped magnetic order is induced, which competes with high-Tc superconducting phase [3].
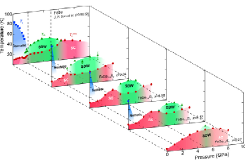
Fig. 1. Temperature-pressure-concentration phase diagram in FeSe1-xSx. The structural (Ts, blue squares), magnetic (Tm, green triangles) and superconducting transition temperatures (Tczero, red circles) are plotted against hydrostatic pressure P and S content x. Ts, Tm and Tczero are defined respectively by the temperatures of upturn, kink and zero resistivity in ρ(T) curves under pressure for x = 0.04, 0.08, 0.12 and 0.17.
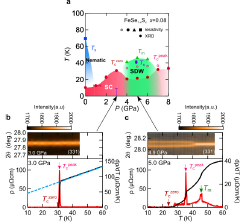
Fig. 2. (a) Temperature-pressure phase diagram for FeSe1-xSx.(x = 0.08) together with Ts determined by the high-pressure synchrotron X-ray diffraction (XRD) in a diamond anvil cell (the purple hexagon with error bars). (b), (c) Temperature dependence of Bragg intensity as a function of 2θ angle is indicated in color scale for 3.0 GPa (b) and 4.9 GPa (c). ρ(T) and dρ/dT are also shown with the same horizontal axis. The red, pink and green arrows indicate Tczero, Tcpeak and Tm, respectively.
Here we report on our high pressure study on FeSe1-xSx in wide ranges of pressure (up to P ~ 8 GPa) and sulfur content (0 < x ≤ 0.17) by the electronic resistivity measurements with a cubic anvil high pressure apparatus in Uwatoko group and X-ray diffraction measurements with a diamond anvil cell in SPring-8 [4].
Our single crystalline samples were synthesized by the chemical vapor transport method. The electric resistivity measurements were carried out for FeSe1-xSx (x = 0.04, 0.08, 0.12, and 0.17). From the anomalies seen in the temperature dependence of the electrical resistivity, the tetragonal-to-orthorhombic structural transition temperature Ts corresponding to the electronic nematic transition, the superconducting transition temperature Tc and the magnetic phase transition temperature Tm are determined, and their pressure dependence is investigated. From the results, we establish a three-dimensional Temperature(T)-pressure(P)-S substitution(x) electronic phase diagram as shown in Fig. 1. As x increases, the pressure-induced magnetic dome shifts to higher pressure and shrinks, while the low-pressure non-magnetic nematic phase shifts to lower pressure and disappears at x ~ 0.17. From this change, we find that the non-magnetic nematic and magnetic phases are completely separated in a low pressure region. To confirm the separation between two phases under pressure, we performed synchrotron X-ray diffraction under pressure for x = 0.08. In Fig. 2 we show (331) Bragg intensity as a function of temperature at 3.0 and 4.9 GPa together with the ρ(T) and dρ/dT data. At 3.0 GPa, no discernible change of the Bragg-peak is observed down to 10 K. At 4.9 GPa, on the other hand, the splitting of the Bragg peak is resolved around Ts ~ 41 K, evidencing the presence of the tetragonal-orthorhombic structural transition. This structural transition is located very close to the magnetic transition at Tm at 5.0 GPa as indicated by the sharp peak in dρ/dT curve. Thus, it is natural to consider that the magnetic phase is accompanied with an orthorhombic structure, similar to the case of the stripe-type magnetic phase in other iron-pnictide superconductors.
By simultaneously tuning chemical and physical pressures, we achieve a complete separation of nematic and antiferromagnetic phases. In between, an extended non-magnetic tetragonal phase emerges, where Tc shows a striking enhancement. The high Tc superconductivity is observed near both ends of the magnetic dome. From this result, the fluctuations of magnetic order are likely to be more relevant to the high-Tc superconductivity in this system.
References
- [1] S-H. Baek et al., Nature Materials 14, 210 (2015).
- [2] S. Hosoi et al., Proc. Natl. Acad. Sci. USA 113, 8139 (2016).
- [3] J. P. Sun et al., Nat. Commun. 7, 12146 (2016).
- [4] K. Matsuura et al., Nat. Commun. 8, 1143 (2017).