Field Evolution of Quantum Critical and Heavy Fermi-liquid Components in the Magnetization of the Mixed Valence Compound β-YbAlB4
Nakatsuji, Sakakibara, Tokunaga, and Kindo Group
Formation of novel quantum phases in the vicinity of a quantum critical point (QCP) has been studied extensively in condensed matter physics for a past few decades. In particular, in the heavy fermion intermetallic systems, a number of prototypical examples of novel phenomena, such as unconventional superconductivity and non-Fermi liquid (NFL) behavior, have been discovered in the vicinity of a magnetic QCP where the magnetic ordering temperature is suppressed to zero.
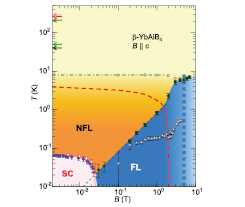
Fig. 1. T-B phase diagram of β-YbAlB4 for the field applied along the c axis. The valence fluctuation scale is shown as pink, red and dark yellow arrows (from top to bottom) at T ~ 200 - 300 K which corresponds to T scale obtained by the X-ray adsorption measurements [7], the coherence peak in ρabm [1] and the peak temperautre in χab at B = 0.1, 7.0 T, respectively. The light and dark green arrows indicate the anomalies in the T derivative of the in-plane resistivity dρab /dT at T ~ 50 K [8] and the peak in the Hall coefficient RH at ~ 40 K [6], respectively. These temperature scales correspond to the effective Kondo lattice temperature for the low T HF behavior. Green filled circles correspond to the peaks in -dχ/dT, which separates the QC and FL regions. Green open circles correspond to the peak temperature scale T* in Δ(-dχ/dT) obtained after subtracting QC components, which sets the onset of the heavy fermion state. Black open circles correspond to TFL determined by ρab [1]. Filled and open diamonds correspond to superconducting phase boundary determined by the SQUID ac susceptibility measurements [9] and the resistivity measurements [2], respectively. Inside the red broken line, the QC scaling is observed. The vertical blue broken line at B ~ 5 T indicates the crossover field above which the HF state is suppressed.
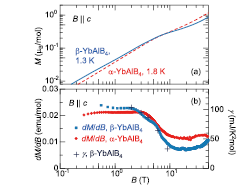
Fig. 2. (a) Magnetization curves of β- and α-YbAlB4 at T = 1.3 and 1.8 K, respectively. (b) dM/dB obtained from the data shown in (a) (the left axis) and the electronic specific heat coefficient γ estimated from the electric specific heat at T = 0.4 K [3] (the right axis).
So far, these studies of quantum criticality (QC) have been restricted mostly to the Kondo lattice systems with integer valence. On the other hand, the first Yb-based heavy fermion superconductor β-YbAlB4 provides a unique example of QC in the strongly mixed valence states [1-4]. Furthermore, the QC cannot be described by the standard theory for the spin-density-wave instability. The divergent magnetic susceptibility along the c axis exhibits T/B scaling in the wide temperature (T) and magnetic field (B) region spanning 3 ~ 4 orders of magnitude. This indicates that the QCP is located just at the zero magnetic field within the experimental resolution of ~ 0.2 mT under ambient pressure [3]. The QC emerging without tuning any control parameter suggests the formation of an anomalous metallic phase.
Here, we discuss the magnetization (M) in β-YbAlB4 in the T range from 0.02 K to 320 K spanning four orders of magnitude to see how it evolves with magnetic field in detail, providing a T-B phase diagram to summarize the various T and B scales for β-YbAlB4 (Fig. 1) [5]. First of all, we examined the possibility of other scaling such as T/(B-Bc)δ scaling with Bc ≠ 0 or δ ≠ 1, and confirmed that the T/B scaling reported in our previous work [3] provides the best quality of the fitting [5].
We further estimated the heavy Fermi-liquid component of M after subtracting the QC component by using the T/B scaling. The obtained heavy Fermi-liquid component exhibits the T and B dependence quite similar to the magnetization of α-YbAlB4, having a peak in -dM/dT at T* ~ 8 K, which is suppressed above B* ~ 5 T. Here, α-YbAlB4 is the locally isostructural polymorph of β-YbAlB4 and is also strongly mixed valent (Yb+2.73 and Yb+2.75 for α- and β-YbAlB4 at 20 K, respectively) [4]. Our observation indicates that the HF behavior in α- and β-YbAlB4 becomes suppressed above B* ~ 5 T corresponding to the small renormalized Kondo scale. This was also confirmed by the analysis of the field dependence of the electronic specific heat coefficient of β-YbAlB4 and the measurements of the magnetization curve up to ~ 50 T for both α- and β-YbAlB4. This suppression of the HF behavior may be related to the recent observation of the field induced anisotropic NFL behavior in α-YbAlB4. In β-YbAlB4, on the other hand, we found no sign of such field induced NFL behavior.
While the effective g-factor estimated from the Zeeman coupling relation kBT* = gμBB* and T/B scaling suggests the effective moment of ~ 2 μB for β-YbAlB4, M at the suppression field B* only reaches a considerably smaller value ~ 0.2 μB. This indicates that only a part of the f moments participates in the zero-field QC or HF behavior, as also suggested by the recent Hall effect measurements [6]. The multiple bands and their topological feature should play an important role in the formation of the low temperature heavy fermion state and quantum criticality in the intermediate valence state.
References
- [1] S. Nakatsuji, K. Kuga, Y. Machida, T. Tayama, T. Sakakibara, Y. Karaki, H. Ishimoto, S. Yonezawa, Y. Maeno, E. Pearson, G. G. Lonzarich, L. Balicas, H. Lee, and Z. Fisk, Nature Physics 4, 603 (2008).
- [2] K. Kuga, Y. Karaki, Y. Matsumoto, Y. Machida, and S. Nakatsuji, Physical Review Letters 101, 137004 (2008).
- [3] Y. Matsumoto, S. Nakatsuji, K. Kuga, Y. Karaki, N. Horie, Y. Shimura, T. Sakakibara, A. H. Nevidomskyy, and Piers Coleman, Science 331, 316 (2011).
- [4] M. Okawa, M. Matsunami, K. Ishizaka, R. Eguchi, M. Taguchi, A. Chainani, Y. Takata, M. Yabashi, K. Tamasaku, Y. Nishino, T. Ishikawa, K. Kuga, N. Horie, S. Nakatsuji, and S. Shin, Physical Review Letters 104, 247201 (2010).
- [5] Y. Matsumoto, K. Kuga, Y. Karaki, Y. Shimura, T. Sakakibara, M. Tokunaga, K. Kindo, and S. Nakatsuji, Journal of the Physical Society of Japan 84, 024710 (2015).
- [6] E. C. T. O’Farrell, Y. Matsumoto, and S. Nakatsuji, Physical Review Letters 109, 176405 (2012).
- [7] Y. H. Matsuda, T. Nakamura, K. Kuga, S. Nakatsuji, S. Michimura, T. Inami, N. Kawamura, and M. Mizumaki, Journal of the Korean Physical Society 62, 1778 (2013).
- [8] Y. Matsumoto, K. Kuga, T. Tomita, Y. Karaki, and S. Nakatsuji, Phys. Rev. B 84, 125126 (2011).
- [9] Y. Matsumoto, K. Kuga, Y. Karaki, T. Tomita, and S. Nakatsuji, Phys. Status Solidi B 247, 720 (2010).