High Field Investigation of Field Induced Phase Transitions in BiCu2PO6
Kindo, Y. Matsuda, and Takeyama Groups
A frustrated two-leg ladder spin-gap system, BiCu2PO6, has recently attracted intense theoretical and experimental interest [1,2]. An application of a magnetic field along the leg direction of the spin ladder (H//b) closes the spin gap at Hc1 ~ 20T and induces a series of field-induced phase transitions at higher magnetic fields [1]. Recent nuclear magnetic resonance (NMR) experiments by F. Casola have observed a triple-peak NMR profile in the intermediate field range from 20 to 35 T, and they have interpreted it as an appearance of an exotic "quantum soliton phase" [2]. However, since the measurements at high magnetic fields are experimentally challenging, detailed researches above 35 T remain scarce. In this context, we have experimentally investigated the field-induced phase-transitions under ultrahigh magnetic fields, i.e. up to 120 T, for three different crystallographic axes, by means of a combination of state-of-the-art techniques including specific heat (Cp), magnetocaloric effect (MCE), magnetization (M), and Faraday rotation (ΘM) [3]. Here we have provided the thermodynamic evidence for the formation and the subsequent collapse of this field-induced soliton lattice.
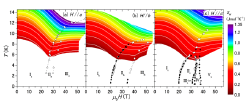
Fig. 1. (a)-(c) Contour map of S(T ,H) for H//a (left), H//b (middle), and H//c (right). The contour plot of SM (T ,H) is constructed through linear interpolation of a series of MCE data. The phase boundaries determined by MCE and specific heat are also shown as open circles and open triangles, respectively. The solid dots are the phase boundaries reported in Ref. [1].
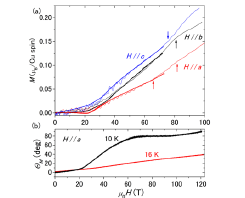
Fig. 2, (a) Magnetization (M) of BiCu2PO6. Thin curves were obtained by using a single turn coil. Thick curves were measured in a 75 T nondestructive pulsed magnet. (b) Field dependence of Faraday rotation angle (ΘM) at 10 and 16 K. The magnetic field was applied along the a axis.
Figure 1 shows the (H,T) phase diagram for BiCu2PO6. The phase boundaries depicted by open triangles and circles were determined by Cp and MCE measurements, respectively. Here, it should be emphasized that the MCE measurement were carried out under adiabatic conditions. Thus, the obtained MCE curves correspond to isentropic temperature changes as a function of the magnetic field, i.e., the entropy remains constant when collecting reversible MCE curves. Using the isentropic curve and specific heat data, we have succeeded to estimate the magnetic entropy (SM), as seen in Fig.1(a)-(c) as color contour plots. We find that the Phase IIb (20 T < H < 35 T // b axis) can be characterized by high entropy, which is subsequently observed to disappear when entering phase IIIb. This observation is in line with a recent study suggesting the formation and the collapse of a soliton lattice [2], which is predicted to have high entropy due to the short-ranged magnetic order.
The magnetization (M) and Faraday rotation (ΘM) shown in Fig. 2 captures further features in the ultrahigh magnetic field region. (i) For H//a, additional weak anomalies in M are observed in fields exceeding 60 T, a kink and a shoulder in M at 65 and 80 T (red arrows), respectively, indicating further field-induced transitions. This finding is also supported by the ΘM measurements as a function of H [Fig. 2(b)]. (ii) When the external magnetic field is applied along the b axis, we can find a broad shoulder in M at 80 T (indicated by a black arrow). For H//c, an upturn at 75 T is evident in M (blue arrow). Although we observe a strongly anisotropic magnetization, the sequence of field-induced transitions continues above 60 T, regardless of field orientation. Our results indicate that BiCu2PO6 undergoes a sequence of novel phase transitions from a quantum soliton lattice to other exotic states under fields up to 120 T.
References
- [1] Y. Kohama, S. Wang, A. Uchida, K. Prsa, S. Zvyagin, Y. Skourski, R. D. McDonald, L. Balicas, H. M. Ronnow, C. Rüegg, and M. Jaime, Phys. Rev. Lett. 109, 167204 (2012).
- [2] F. Casola, T. Shiroka, A. Feiguin, S. Wang, M. S. Grbić, M. Horvatić, S. Krämer, S. Mukhopadhyay, K. Conder, C. Berthier, H.-R. Ott, H. M. Rønnow, Ch. Rüegg, and J. Mesot, Phys. Rev. Lett. 110, 187201 (2013).
- [3] Y. Kohama, K. Mochidzuki, T. Terashima, A. Miyata, A. DeMuer, T. Klein, C. Marcenat, Z. L. Dun, H. Zhou, G. Li, L. Balicas, N. Abe, Y. H. Matsuda, S. Takeyama, A. Matsuo, and K. Kindo, Phys. Rev. B 90, 060408(R) (2014).