Pressure Induced Superconductivity in CrAs
Uwatoko Group
The transition-metal pnictide compounds MX (M = 3d transition metal, and X = P, As, Sb) have been studied since 1960s as a paradigm for studying the intimated relationship between magnetism and structural parameters of metallic magnets. Among these compounds, CrAs, stands out due to its peculiar magnetic and structural transitions as detailed below. At room temperature, CrAs adopts the orthorhombic MnP-type structure with lattice parameters a = 5.649Å, b = 3.463 Å, and c = 6.2084 Å. Upon decreasing temperature to TN = 265 K, it displays an isostructural, first-order transition with discontinuous changes of lattice parameters; the most dramatic change is a sudden jump of lattice constant b by ~ 3% with a net volume expansion. Neutron powder diffraction measurements revealed that this structural transition is accompanied by a double helical antiferromagnetic order with the localized moments of ~1.7 μB/Cr lying essentially within the ab plane and propagating along the c axis. The acquisition of localized Cr moment below TN has been rationalized as an itinerant- (or collective) to localized-electron transition due to the sudden elongation of Cr-Cr separation along the b direction. By monitoring the resistivity anomaly at TN, subsequent high-pressure studies have shown that this transition is very sensitive to external pressure and it can be suppressed completely at ~ 6 kbar. These observations seem to be consistent with the scenario of localized-to-itinerant transition since pressure tends to reduce the b axis and also the volume. Thus, CrAs provides us a unique opportunity to explore the localized-to-itinerant transition as well as the possible quantum critical point by applying minute pressure.
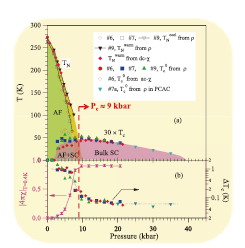
Fig. 1. (a) Temperature-pressure phase diagram of the Pressure-Induced Superconductivity in the antiferromagnetic CrAs. The superconducting transition temperature Tc has been scaled by a factor of 30 for clarity. (b) The superconducting shielding fraction at 0.4 K, |4πχ|T=0.4K, and the superconducting transition temperature width, ΔTc, as a function of pressure. The symbols of circle (red), square (blue), and triangle (green) in (a, b) represent three independent samples with residual resistivity ratio RRR = 240, 327, and 250, respectively.
We measured the resistivity ρ(T) and ac magnetic susceptibility χ’(T) of a number of CrAs single crystals under various hydrostatic pressures up to 21.4 kbar in a wide temperature range from 300 K down to 70 mK. CrAs single crystals were grown out of the Sn flux. We found that CrAs becomes superconducting when its coupled magnetic/structural transition is suppressed by pressure, and CrAs is the first superconductor among the Cr-based compounds. The phase diagram of CrAs is shown in Fig. 1(a). The major findings include: (i) TN decreases progressively with pressure and vanishes completely at a critical pressure Pc ≈ 9 kbar; (ii) the transition at TN remains to be first order until disappears and the thermal hysteresis enlarges with pressure; (iii) the superconducting state emerges upon the application of pressure, and bulk superconductivity with a volume fraction over 90% develops eventually when TN vanishes completely above 9 kbar, (Fig 1(b); (iv) the established T-P phase diagram resembles those of unconventional superconductors such as the heavy-fermion and iron-based superconductors; and Tc(P) displays a dome shape with a broad maximum around 10±2 kbar; (v) the superconducting state can deteriorate if the level of impurity and/or disorder in the sample is high, a key signature for non-s-wave pairing symmetry; (vi) the normal-state resistivity was found to deviate from the Fermi-liquid behavior in a large pressure range. The above observations indicated that the observed superconductivity in CrAs should fall into the unconventional category [1].
References
- [1] W. Wu, J.-G. Cheng, K. Matsubayashi, P. P. Kong, F. K. Lin, C. Q. Jin, N. L. Wang, Y. Uwatoko, and J. L. Luo; Nature Communications 5, 5508 (2014).