Spin Dynamics in Novel Rare-Earth
Based Single-Molecule Magnets
Yamamuro Group
Single-molecule magnets (SMMs) are a class of metal-organic compounds in which each constituent molecule, containing magnetic atoms, possesses a giant and isolated resultant spin. When the giant spin exhibits easy-axis magnetic anisotropy (D < 0), the molecule has a ground state with Sz = ± S, and the potential barrier between the states can be simply described as DSz2. The presence of barrier yields a slow relaxation of the magnetization reversal that is characteristic of SMM. To date, SMMs containing transition metal atoms such as Mn, Fe, and Ni, have been intensively studied. Recently, a new series of rare-earth based SMMs was discovered and attracts much attention. Because of large contribution of angular momenta, lanthanide complexes can become SMMs containing only one or two magnetic atoms, being simpler than the transition metal SMMs consisting of many magnetic atoms and suitable for fundamental studies. Besides, the energy scale of lanthanide SMMs is expected to be larger and they can be good candidates for magnetic devices. Now we work on dinuclear SMMs consisting Tb3+ and Cu2+ ions. Interestingly, the system switches from SMMs to non-SMMs by a slight structural modification around the Tb3+ ions [1]. In order to investigate their spin states and energy scheme, we have performed inelastic neutron scattering experiments on the AMATERAS spectrometer newly built at J-PARC.
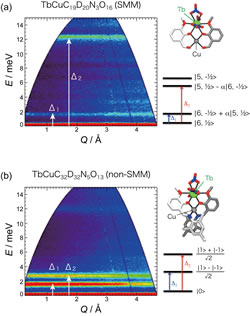
Fig.1. Dynamical structure factors as functions of energy and momentum transfer for (a) complex 1 and (b) complex 2 taken at T = 6 K. Redder color denotes higher intensity. Molecular structures and energy scheme are also shown.
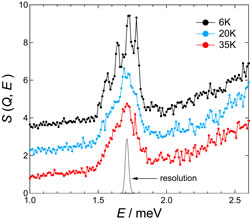
Fig.2. Energy dependence of dynamical structure factors at T = 6, 20, and 35 K. Solid line represents the instrumental resolution.
Figure 1 shows the dynamical structure factor, S(Q,E), as functions of energy (E) and momentum transfer (Q) for TbCuC19D20N3O16 (complex 1 : SMM) and TbCuC32D32N5O13 (complex 2 : non-SMM). The S(Q,E) map of complex 1 is much different from that of complex2. Taking account of scattering intensity and the selection rule, Δ S = ± 1, we determined the energy scheme as shown in Fig. 1. In complex 1, the excitation with E = 1.7 meV corresponds to the transition from |Jz = 6, Sz = 1/2> to |6, -1/2>, while that with E = 12.3 meV from |6, 1/2> to |5, 1/2>, where Jz(Sz) is z-component of Tb3+ (Cu2+) magnetic moment. Assuming the simple spin Hamiltonian, H = DJz2 + Jex J·S, D and Jex are estimated to be -1.1meV and -0.29 meV respectively. Note that the Tb3+ moment and the Cu2+ spin are coupled by the Heisenberg interaction. This is justified by the fact that the scattering intensity varies as sin(Qd)/Qd, where d corresponds to the distance between Tb and Cu ions. It gives rise to the hybridization between |6, -1/2> and |5, 1/2> states.
On the other hand, for complex 2, it is the most plausible scheme that the ground state is |Jz = 0> and the observed excitations around 2 meV corresponds to the excitations from |Jz = 0> to (|Jz = 1> ± |Jz = -1>)/√2. Here, we neglect the contribution from the Cu2+ spin because the term, J·S, must be small in this case. Assuming H = DJz2 + E(Jx2 - Jy2), we obtained D = 2.2 meV and E = 0.03 meV. Therefore, the magnetic anisotropy of Tb3+ in complex 1 (SMM) is easy-axis type (D < 0), while that in complex 2 (non-SMM) easy-plane one (D > 0).
Now let us discuss the mechanism of magnetization reversal in complex 1. The activation energy estimated by the ac-magnetic susceptibility measurements is ca. 1.5meV [1] which is close to the energy difference between |6, 1/2> and |6, -1/2>. We speculate the magnetization reversal occurs through the quantum tunneling between |6, -1/2> and |-6, 1/2> states. However, the Hamiltonian mentioned above cannot cause the hybridization between these states. Our neutron scattering experiments under the high-resolution condition revealed that the peak at 1.7meV splits as shown in Fig. 2. This peak-splitting may result from the hyperfine interaction between the nuclear spin and electron spin and orbital magnetic moments. We predict that the hyperfine interaction plays an important role in the hybridization states that yield the tunneling process of magnetization reversal.
References
- T, Kajiwara, M. Nakano, S. Takaishi, and M. Yamashita, Inorg. Chem. 47, 8604 (2008).