Mechanism of Superconductivity in Graphite Intercalation Compounds (GICs)
Takada Group
In the study of the superconducting transition temperature Tc by ab initio calculations, the ultimate goal is to predict Tc by using only the information on elements composing superconductors. A less ambitious and yet very important goal is to make an accurate estimation of Tc directly from a microscopic Hamiltonian (in particular, without resort to the concept of the Coulomb pseudopotential μ*), which helps us not only better understand the mechanism of superconductivity but also obtain valuable hints to synthesize a room-temperature superconductor.
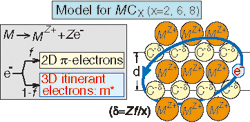
Fig.1. In the GICs denoted by MCx, the metal element M is ionized into MZ+ with Z the valence. The valence electrons released from M go into either the two-dimensional (2D) π-bands on the graphite layers or the three-dimensional (3D) interlayer band with the branching ratio of f : (1 - f). The 3D electrons with the effective mass m* superconduct with the formation of the Cooper pairs mediated by the polarization waves of ions.
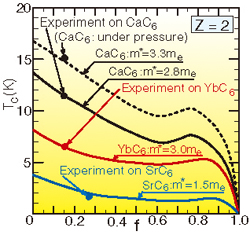
Fig. 2. Calculated Tc as a function of the branching ratio f. The calculation is done from first principles based on the model in Fig. 1 with using the band mass of the 3D electrons for m*. The experimental result of Tc for each GIC is plotted at the value of f determined by the band-structure calculation.
In pursuit of the latter goal, a proposal was made in 1978 for a scheme of calculating Tc directly from the dynamic electron-electron effective interaction V(q,ω), including both the phonon-mediated attraction and the Coulomb repulsion on the same footing [1]. The scheme was successfully applied to the n-type semiconducting SrTiO3 in which polar-coupled phonons leading to the stress-induced ferroelectrics were clarified to play a crucial role [2]. This success has assured us of both usefulness of this scheme and adequacy to employ the random-phase approximation in determining V(q,ω) in the polar-phonon mechanism. In 1982 this scheme was also successfully applied to the alkali-doped GICs such as KC8 and RbC8 with Tc in the range 0.01-0.1K by adopting such a model for the GICs as schematically shown in Fig. 1 [3].
In 2005, a breakthrough occurred in the field of the GIC superconductors. By changing alkali dopants into alkaline-earth ones in synthesizing the GICs, Tc was found to be very much enhanced. In CaC6, for example, Tc=11.5 K, which was increased further to 15.1 K under pressures, and in YbC6, Tc=6.5 K. Note that Tc in CaC6 is about a hundred times higher than that in KC8, though the ion masses are about the same.
In order to check whether the same model in Fig. 1 applies to the newly-synthesized alkaline-earth-doped GIC superconductors or not, we have recently calculated Tc based on exactly the same scheme [4]. The parameters in the model have been changed appropriately for the new superconductors; for example, we have used Z=2 instead of Z=1, m*~3me (due to the mixing of d electrons with the interlayer band) instead of m*=me (me: the mass of a free electron) and f = 0.16 (reflecting the stronger attractive potential of M2+ for the interlayer band) instead of f = 0.6. As shown in Fig. 2, we find that our calculations reproduce all the experimental results quite well, implying that our model must be a standard one representing all GIC superconductors with Tc ranging more than three orders of magnitude. Our calculations also reveal that the key parameters to control Tc are Z and m*; the difference in Tc by two orders between KC8 and CaC6 can be accounted for by (1) doubling Z, which enhances Tc by one order, and (2) tripling m*, which also enhances Tc by one order.
Encouraged by this success and with confidence in the predictive power of our standard model, we have estimated the optimum Tc in the GIC superconductors to find that Tc may become much higher than 10K, if M is chosen as Ti or V. A further enhancement of Tc, which might enter the range 50-100K, can be expected, if two or more kinds of Ms are suitably intercalated, so that both m* and Z are made large enough and the polar-phonon energy is made as large as possible.
References
- Y. Takada, J. Phys. Soc. Jpn. 45, 786 (1978).
- Y. Takada, J. Phys. Soc. Jpn. 49, 1267 (1980).
- Y. Takada, J. Phys. Soc. Jpn. 51, 63 (1982).
- Y. Takada, J. Phys. Soc. Jpn. 78, 013703 (2009).