Kondo Signature in Heat Transfer via a Local Two-State System
Kato Group
Heat and electric transport have several similarities as well as dissimilarities. Fourier's law in heat transport corresponds to Ohm's law in electric transport, and these laws are commonly categorized as diffusive transport. Ballistic transport leads to the quantization of conductance in electric as well as heat transport. The conductance quantum was measured in mesoscopic electric conduction in 1988, and much later, the version of heat transport was also measured [1]. Recently, the concept of thermal diode has also been discussed, and an experiment has been conducted for demonstrating this [2]. Recent progress in transport studies strongly indicates that heat transport analogue exists for many categories of electric transport.
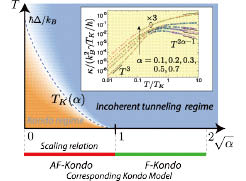
Fig. 1. (Main panel) Two different regimes expected in the spin-boson model. (Inset) The thermal conductance calculated by the quantum Monte Carlo method is shown as a function of the temperature.
We have studied the Kondo effect in heat transport via a local two-state system [3]. We consider a two-state system coupled to phonon environments. The two-state system is, for example, obtained from truncation from a symmetric double-well system with a small tunneling matrix element Δ. The present system is described by the spin-boson Hamiltonian with Ohmic dissipation. The cut-off energy of the phonon bath and the dimensionless system-environment coupling are denoted with ωc and α, respectively. It is known that the spin-boson model can be mapped onto the Kondo model with anisotropic exchange coupling. In order to study the Kondo-like effect in the phonon systems, we have derived the exact formula of thermal conductance, and have evaluated it by the quantum Monte Carlo method.
Summary of the results is shown in Fig. 1. The main figure shows the Kondo regime and the incoherent tunneling regime in the space of temperature T versus dimensionless coupling strength α. At the bottom of the main figure, mapping onto corresponding anisotropic Kondo model is shown: the antiferromagnetic Kondo (AF-Kondo) and the ferromagnetic Kondo (F-Kondo) region are respectively mapped onto the region of 0<α<1 and 1<α<4 in the spin-boson Hamiltonian. Heat transport via the Kondo effect is realized in the Kondo regime (the orange region).
In the inset of the figure, we show temperature dependence of the thermal conductance κ calculated by the quantum Monte Carlo method. Different legends in the graph denote with different ratios of Δ/ωc. As seen in the figure, the data for a fixed α fall onto one curve by appropriate scaling of the temperature and the thermal conductance with the Kondo temperature TK. This behavior is characteristic of the Kondo effect. Below the Kondo temperature, conductance follows the universal temperature dependence proportional to T3. The obtained thermal conductance is much larger than the one expected from stochastic transition of the two-state system [4]. This is a manifestation of strong correlation between system and reservoirs, which is analogous to the Kondo effect in electric transport. On the other hand, at temperatures higher than the Kondo temperature TK, the thermal conductance becomes proportional to T2α-1. This behavior is understood by a simple approximation based on the Fermi's golden rule. We note that the thermal conductance is always proportional to T2α-1 for α>1 since the Kondo temperature is zero.
In summary, we have investigated the Kondo effect in thermal transport via a local two-state system, by utilizing the spin-boson model with ohmic dissipation. We hope that our study motivates further research on low-energy heat transfer via local systems. Other types of dissipation (superohmic/subohmic dissipation) and far-from-equilibrium effect will be intriguing subjects in this direction.
References
- [1] B. J. Wees et al., Phys. Rev. Lett. 60, 848 (1988); K. Schwab et al., Nature (London) 404, 974 (2000); H.-Y. Chiu et al., Phys. Rev. Lett. 95, 226101 (2005).
- [2] N. Li, J. Ren, L. Wang, G. Zhang, P. Hänggi, and B. Li, Rev. Mod. Phys. 84, 1045 (2012); C. W. Chang, D. Okawa, A. Majumdar, and A. Zettl, Science 314, 1121 (2006).
- [3] K. Saito and T. Kato, Phys. Rev. Lett. 111, 214301 (2013).
- [4] T. Ruokola and T. Ojanen, Phys. Rev. B 83, 045417 (2011).